DOI: 10.12809/hkmj176875
© Hong Kong Academy of Medicine. CC BY-NC-ND 4.0
MEDICAL PRACTICE
Hyperbaric oxygen therapy: its use in medical
emergencies and its development in Hong Kong
Joe KS Leung, MHM (UNSW), FHKAM (Emergency
Medicine)1; Rex PK Lam, MPH, FHKAM (Emergency Medicine)2
1 Accident and Emergency Department,
Pamela Youde Nethersole Eastern Hospital, Chai Wan, Hong Kong
2 Emergency Medicine Unit, Li Ka Shing
Faculty of Medicine, The University of Hong Kong, Pokfulam, Hong Kong
Corresponding author: Dr Joe KS Leung (leungksj@ha.org.hk)

Abstract
Hyperbaric oxygen therapy is widely accepted as
life-saving treatment for decompression illness. Yet its use in acute
carbon monoxide poisoning has remained controversial because of
inconsistent findings in clinical trials. Hyperbaric oxygen therapy has
an adjunctive role in managing gas gangrene, necrotising soft-tissue
infection, and crush injury, as supported by case series. Several cases
have been reported in the literature detailing the use of hyperbaric
oxygen therapy in patients with severe anaemia in whom blood transfusion
is not possible. Today, use of hyperbaric oxygen therapy in Hong Kong is
limited by low awareness among physicians and patients, a lack of
service access, and inadequate hospital and critical care support for
the existing non-hospital facility. The recent introduction of a
hospital-based facility is expected to benefit more patients for whom
hyperbaric oxygen therapy is appropriate. This article reviews the
mechanistic basis of and emerging scientific evidence to support the use
of hyperbaric oxygen therapy in a number of acute medical emergencies,
as well as the past and future development of hyperbaric oxygen therapy
in Hong Kong.
Introduction
Hyperbaric oxygen therapy (HBOT) is not a new
treatment modality. Medical use of alterations in ambient pressure can be
traced back to 1662, when Henshaw built the first hyperbaric chamber
(Domicilium), a century before the discovery of oxygen.1 The beneficial effects of increased pressure as therapy
for decompression illness (DCI) became evident more than 100 years ago,
leading subsequently to the discovery of a synergy between pressure and
high oxygen levels that provides the physical and biological basis of what
is now known as ‘hyperbaric oxygen therapy’. This therapy is now used in a
wide range of medical conditions and hyperbaric medicine has emerged as a
clinical discipline in many countries. Today, the use of HBOT in Hong Kong
is still limited because of low awareness among physicians and patients,
and lack of access to an HBOT facility, especially in the hospital
setting. In this article, we review the mechanistic basis of and evidence
supporting the use of HBOT in five selected medical emergencies, as well
as the past and future development of HBOT service in Hong Kong.
Clinical use of hyperbaric oxygen therapy
Defined by the Undersea and Hyperbaric Medical
Society (UHMS), HBOT is “an intervention in which an individual breathes
near 100% oxygen intermittently while inside a hyperbaric chamber that is
pressurised to greater than sea level pressure (1 atmosphere absolute, 1
ATA)”.2 The pressure must exceed
1.4 ATA for clinical purposes and its application must be systemic to the
patient’s body—that is, topical application is not considered HBOT.
Hyperbaric chambers are classified according to occupancy. Monoplace
chambers are designed for a single person and generally pressurised with
100% oxygen. Multiplace chambers are intended for concurrent use by more
than one patient and are pressurised with air, with oxygen given via a
face-mask, hood tent, or endotracheal tube.
Whereas pressure per se has some therapeutic effect
in bubble-related diseases, the biological essence of HBOT is extreme
hyperoxia, enabled via increased pressure. Under pressure, the physical
behaviour of gases is governed by gas laws; those that are fundamental to
understanding HBOT are summarised in Table 1.3 Of
note, HBOT has complex biological effects that extend beyond increasing
the amount of dissolved oxygen. Over the years, different additional
mechanisms and applications have been reported in the literature.
At present, the UHMS approves 14 clinical
indications for HBOT (Box).2
Different treatment protocols (known as treatment tables), consisting of
different combinations of pressure and durations of oxygen and air
breathing, have been devised for different conditions. The incidence of
adverse effects is reported to be between 5 and 50 per 1000 HBOT
exposures, depending on the indication, clinical setting, treatment
protocol, and patient’s conditon.4
The adverse effects of and contra-indications to HBOT are summarised in Tables 2 and 3, respectively. Because of limited space, this
article focuses on five acute medical conditions encountered in the
emergency setting: DCI, carbon monoxide (CO) poisoning, acute infections,
acute crush injuries, and severe anaemia. Readers are advised to refer to
the relevant literature for clinical indications not covered in this
article.
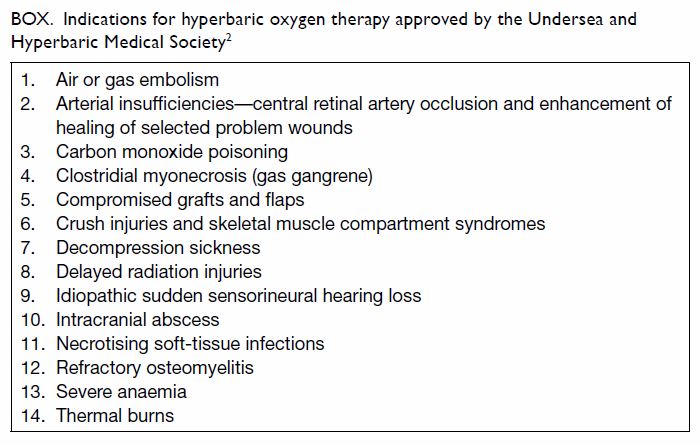
Box. Indications for hyperbaric oxygen therapy approved by the Undersea and Hyperbaric Medical Society2
Decompression illness
Decompression illness is caused by an acute
reduction in ambient pressure leading to formation of intravascular or
extravascular gas bubbles. Gas embolism and decompression sickness (DCS)
are the two major forms.
Gas embolism occurs when gas enters the arterial
(arterial gas embolism [AGE]) or venous (venous gas embolism [VGE])
circulation. Diving and iatrogenic gas embolism are the two main causes.5 Diving embolism is precipitated by
rapid ascent, breath-holding, or the presence of pre-existing lung
pathology. Overexpansion of the lung during ascent causes barotrauma,
alveolar or small airway rupture, and air entry into the pulmonary veins
followed by air transit to the arterial circulation. Iatrogenic gas
embolism can occur as a complication of a variety of invasive medical
procedures, including central line placement, cardiopulmonary bypass,
laparoscopic surgery, and a range of open surgical procedures.6 In general, VGE is relatively better tolerated because
gas in the venous system is filtered by the pulmonary vessels. Venous gas,
however, can migrate to the arterial system when there is right-to-left
shunting (eg, through a patent foramen ovale) or pulmonary filtration
overload (large amount of bubbles), causing ‘paradoxical embolism’.7
In AGE, the gas bubbles can occlude any end artery,
directly inducing distal tissue ischaemia. However, most AGE pathology
probably accrues from damage to the endothelium, triggering a cascade of
haemostatic and inflammatory responses, endothelial leak and vasogenic
oedema, and ischaemia-reperfusion injury.5
6 Clinical manifestations, usually
sudden in onset (or for divers, within a few minutes of surfacing), depend
on the location of the gas embolus and the quantity of gas. Of note, AGE
can result in severe morbidity or even death if it involves coronary or
cerebral arteries. Coronary artery emboli can lead to myocardial
ischaemia, cardiac failure, dysrhythmia, or even cardiac arrest. Cerebral
AGE can present as a stroke with focal neurological deficits, loss of
consciousness, seizure, or even coma.
Decompression sickness occurs when the rate of
ambient pressure reduction exceeds that of inert gas (mainly nitrogen)
washout from tissue. When a diver ascends following a period of time
underwater, the partial pressure of dissolved inert gas in capillaries and
tissues is greater than the ambient pressure (Henry’s Law and Dalton’s
Law) and off-gassing occurs. If supersaturation results, bubbles form in
venous blood and/or tissues. The reported threshold dive depth for DCS is
about 6 m but problems arise only after very prolonged times at such
shallow pressures.8 Such sickness
is very uncommon after diving to depths of less than 10 m. The risk is
also affected by multiple factors such as immersion (vs dry hyperbaric
chamber exposure), exercise, and temperature. In DCS, extravascular
(autochthonous) bubbles cause mechanical distortion of tissues, leading to
pain or dysfunction, depending on the tissue involved; intravascular
bubbles cause a VGE that can arterialise.9
Decompression sickness has a wide range of potential manifestations that
begin minutes to days after surfacing, including constitutional symptoms
such as malaise; joint pain that commonly involves the knees and
characteristically improves on local tissue pressure; mild neurological
symptoms, such as numbness or paraesthesias; and skin rash (livedo
reticularis). Severe cases can present with cardiopulmonary collapse, loss
of consciousness, incomplete or complete spinal cord paresis, or severe
vestibular dysfunction.
Diagnosis of DCI is primarily based on clinical
findings. Clinicians should be aware of the possibility of AGE or DCS when
patients present with compatible symptoms and a history of recent diving.
The possibility of AGE should be considered in any case of sudden-onset
clinical deterioration after high-risk medical procedures. Differentiation
between AGE and DCS in divers may be difficult but is not necessary when
selecting patients for recompression therapy.9
Supportive treatment is the mainstay of
pre-hospital and initial emergency treatment for DCI. High-flow oxygen is
used to correct hypoxia and to create a diffusion gradient from tissue to
alveolar gas for the egress of nitrogen and other gases from the bubbles.
For both AGE and DCS, recompression with HBOT is widely accepted as the
definitive and potentially life-saving treatment despite a lack of
randomised controlled trials (RCTs) in humans. Its use is supported by
more than 100 years of clinical experience, rigorous mechanistic and
outcomes-based research in animal models and human volunteers. The initial
response to therapeutic pressurisation is in accordance with Boyle’s
Law—at 2.8 ATA pressure; bubble volume is immediately reduced by
two-thirds.10 Hyperoxia corrects
tissue hypoxia and, by minimising blood nitrogen, maximises the diffusion
gradient from the embolised gas to circulating plasma, thus optimising
off-gassing. Furthermore, HBOT has anti-oedema and anti-inflammatory
effects in acute injury, in particular inhibiting neutrophil adhesion to
blood vessels, thus reducing reperfusion injury. The therapy is associated
with significant improvement in the majority of patients with AGE.9 11 12 Of note, HBOT should be initiated early once the
patient is stabilised—the UHMS recommends 100% oxygen at 2.8 ATA, with
treatment repeated until symptoms completely resolve or there is no
further improvement, typically after no more than five to ten treatments.2 13
Acute carbon monoxide poisoning
Hyperbaric oxygen therapy has been used in a
variety of acute poisoning settings, including those caused by CO,
methylene chloride, hydrogen sulphide, and carbon tetrachloride; gas
embolism resulting from hydrogen peroxide ingestion; and
methaemoglobinaemia.14 This
article focuses on CO poisoning, as it remains a major cause of
non-medicinal poisoning death15
and often results in persistent or delayed neurological sequelae.16
The pathophysiology of CO poisoning is complex and
readers are referred to excellent reviews by Weaver16 and Roderique et al17
for details. In brief, CO causes tissue hypoxia by forming
carboxyhaemoglobin (COHb) and shifting the oxyhaemoglobin dissociation
curve to the left. It also binds to various haem proteins, impairs
mitochondrial function, causes release of nitric oxide and free radicals,
and triggers inflammation through a myriad of mechanisms independent of
hypoxia.16 17 18 19
Oxygen therapy is the standard treatment. It works
by reversing hypoxia, competing with CO for haemoglobin binding, and
shortening the half-life of COHb (from 320 min in room air to about 70 min
with 100% oxygen at 1 ATA); HBOT further reduces its half-life to 20 min
(100% at 2.5 ATA), and increases the amount of dissolved oxygen in the
plasma.20 Recent studies have
shown that HBOT also restores mitochondrial function,21 reduces brain lipid peroxidation,22 and inhibits the CO-induced inflammatory response by
inhibiting β2 integrin–mediated neutrophil adhesion to brain
microvasculature and by inhibiting lymphocyte sensitisation to myelin
basic protein.23 24 25
Hyperbaric oxygen therapy was first used for CO
poisoning in 196026 but has
remained controversial owing to the conflicting results of RCTs of its
effect on delayed neurological sequelae. These are summarised in Table
4.27 28 29 30 31 32 A Cochrane review in 2011 that involved six RCTs and
1361 participants showed that HBOT does not have a significant benefit in
a pooled random-effects meta-analysis (odds ratio for neurological
deficits, 0.78; 95% confidence interval, 0.54-1.12). The reviewers
cautioned that the “significant methodologic and statistical
heterogeneity” and “design or analysis flaws” of the included trials
warrant cautious interpretation of the results.33
The American College of Emergency Physicians, on the basis of a systematic
literature review, stated that “It remains unclear whether [hyperbaric
oxygen therapy] is superior to normobaric oxygen therapy for improving
long-term neurocognitive outcomes” in CO-poisoned patients.34 Nonetheless, a recent population-based retrospective
cohort study in Taiwan involving 7278 patients showed that HBOT was
associated with reduced mortality in patients with CO poisoning after
adjusting for covariates, especially in those who were younger than 20
years and those with acute respiratory failure.35
These findings add weight to the argument in support of HBOT use in CO
poisoning.
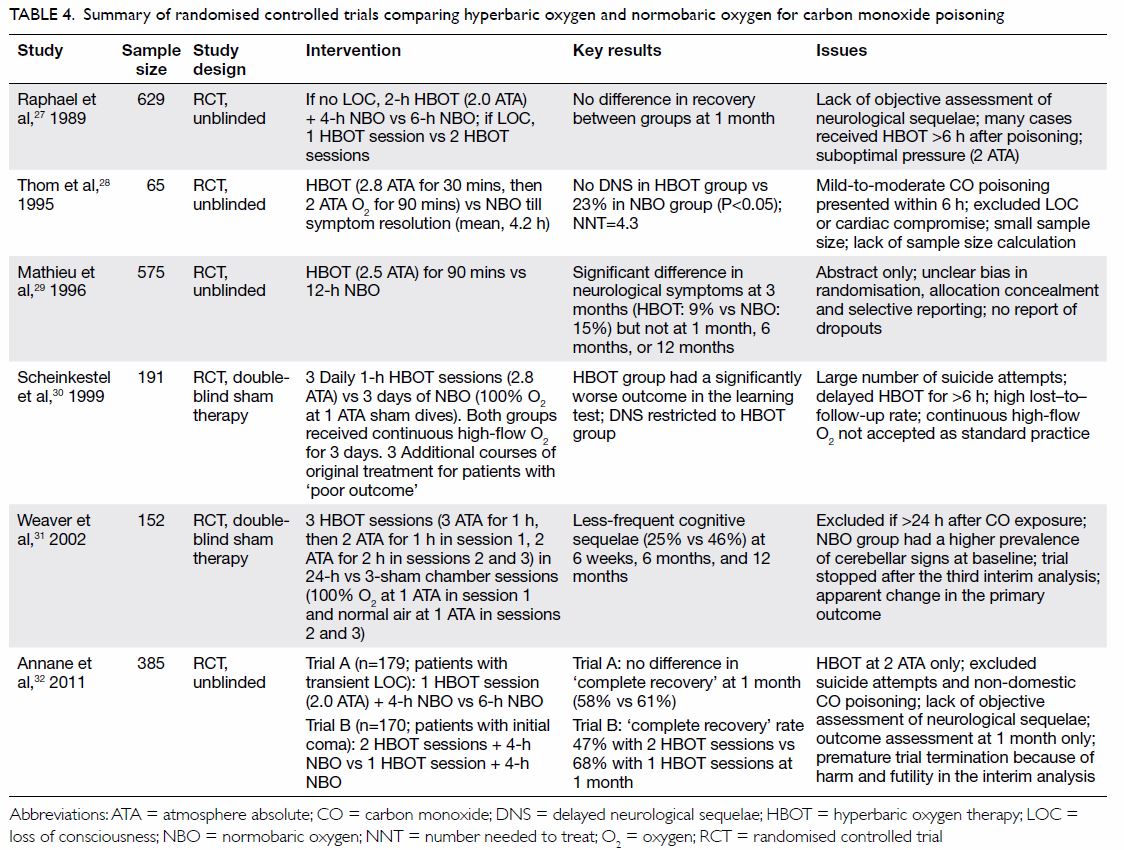
Table 4. Summary of randomised controlled trials comparing hyperbaric oxygen and normobaric oxygen for carbon monoxide poisoning
Yet, the threshold for HBOT use for CO poisoning
varies across different centres36
and uncertainties exist regarding the optimal chamber pressure, number and
frequency of sessions, and time window after CO poisoning. In particular,
pregnant women pose special challenges as they are at high risk of adverse
effects from both CO and HBOT, although information is lacking because
they are excluded from most prospective trials. In view of the devastating
fetal outcomes of maternal CO poisoning, such as stillbirth and damage to
and anatomic malformation of the fetal central nervous system, COHb
thresholds for HBOT are often set lower for pregnant patients (COHb,
15%-20%) and HBOT is often considered indicated when there is evidence of
fetal distress.14 Clinical
experience in Russia supports the contention that HBOT is safe during
pregnancy,37 38 but its benefit in averting CO-related adverse fetal
outcomes is unclear.14 In
CO-poisoned children, indications for HBOT are similar to those for
adults, although they have not been evaluated systematically.39 Studies have reported that HBOT has been used safely
in paediatric patients39 40 but there are special paediatric considerations.
Readers are referred to the review by Liebelt41 for further information.
Before more convincing evidence is available,
clinicians are advised to weigh the benefits, risks, and costs of HBOT
carefully on a case-by-case basis when making a decision about HBOT for
CO-poisoned patients. It is commonly suggested that HBOT should be
reserved for (1) situations where there are indicators of higher-severity
CO poisoning, such as loss of consciousness, abnormal neurological signs,
cardiovascular dysfunction, or severe acidosis; (2) patients older than 35
years; (3) prolonged exposure (eg, >24 hours) or high COHb level (eg,
≥25%16). The most recent UHMS
guidelines nevertheless recommend that HBOT be considered for all cases of
acute symptomatic CO poisoning, given the lack of predictive factors for
poor long-term outcomes or for which patients might receive the greatest
benefit from HBOT.2
Acute infections
Hyperbaric oxygen therapy is directly bactericidal
and/or bacteriostatic to anaerobes, facultative anaerobes, and many
aerobes as a result of bacterial intolerance of the excess oxygen radicals
induced by HBOT. The therapy improves tissue oxygenation, maximises
oxygen-dependent phagocytic function, reduces tissue oedema, and
potentiates uptake and/or action of various antimicrobial drugs, including
the aminoglycosides, fluoroquinolones, and vancomycin.42 The therapy has been used as an adjunct in a variety
of life-threatening bacterial infections—in particular, those with
associated tissue necrosis such as gas gangrene, necrotising fasciitis,
and other necrotising soft tissue infections (NSTIs).
Gas gangrene is a rare but fulminant infection that
is most commonly caused by Clostridium perfringens and germinates
in devitalised and hypoxic tissue. The lethal α-toxin produced by the
organism causes rapidly progressing liquefactive myonecrosis. Hyperbaric
oxygen therapy is bactericidal to C perfringens43 and inhibits toxin production.44 Experimental studies and case series support the use
of HBOT as an adjunct to surgery and antibiotic therapy for gas gangrene.42 Three sessions of HBOT at 3 ATA
for 90 min should be given in the first 24 h, followed by twice-daily
treatments for the next 2 to 5 days, until infection control is achieved.2 45
Other forms of NSTI, often polymicrobial, are often
both life- and limb-threatening. Early HBOT adjunctive to surgery and
antibiotic therapy has been associated with improved survival and limb
salvage,46 47 48 although
several other small case series have suggested no benefit.49 50 In a
large case series that involved 1583 NSTI cases in 14 US centres with
their own facilities, HBOT was associated with increased survival and
fewer complications in the sickest group of patients.51 In heterogeneous, high-acuity, and relatively
uncommon conditions like NSTI, where there is no RCT support for any
particular therapeutic strategy and none is likely, clinicians must base
their decision-making on evidence from observational studies supported by
interpretation of known pathophysiology and therapeutic mechanisms, as
well as from any relevant animal data.52
Hyperbaric oxygen therapy is mechanistically attractive and supported by
what appear to be good outcomes from experienced centres that routinely
use HBOT. It should be considered in patients with serious NSTI, provided
that referral for such treatment does not defer aggressive surgery and
antibiotic therapy. The recommended hyperbaric oxygen protocol is 2.0 to
2.5 ATA for 90 min twice daily until the infection is controlled.2 45
In addition to necrotising bacterial infections,
there are reports supporting HBOT use in treating intracranial abscess,
actinomycosis, and mucormycosis in immunocompromised patients.45 Diabetic foot infections, refractory osteomyelitis,
and certain implant infections are also important indications for HBOT,
but they are outside the scope of this article.
Acute crush injuries and severe anaemia
Crush injury is a spectrum of injury ranging from
minor contusions to limb-threatening damage. The energy of trauma can
cause damage to multiple tissues. Damage to the microvasculature causes
self-perpetuating fluid transudation, tissue oedema, interstitial
bleeding, stasis, tissue hypoperfusion, and hypoxia. Compartment syndrome
occurs when the tissue fluid pressure within a skeletal muscle compartment
exceeds the capillary perfusion pressure to the muscle and nerves in the
compartment. Hyperbaric oxygen therapy works by interrupting the
oedema-ischaemia vicious cycle. It induces inflow vasoconstriction and
reduces tissue oedema, thus improving microcirculatory blood flow. It also
improves tissue oxygen delivery, which is essential in multiple
oxygen-dependent host responses to trauma and infection, mitigates
reperfusion injury, and enhances wound healing.53
The use of HBOT in crush injury is supported by one
small RCT that showed the effectiveness of HBOT in improving wound healing
and reducing repetitive surgery, especially in older patients with
Gustillo grade III soft-tissue injuries.54
A systematic review of nine studies involving 150 patients with crush
injury showed that HBOT is likely to be beneficial if administered early.55 A larger and more rigorous RCT
on open tibial fractures with severe associated soft-tissue injury will be
published in the near future.56
For compartment syndrome, no RCT has been published, but the use of HBOT
is supported by animal studies and small case series.53 The treatment regimen varies depending on the type of
injury, ranging from 2 to 2.4 ATA for 90 min for two or more treatments a
day to 120 min for a single daily treatment.2
53
There are situations in which blood transfusion is
not possible for major blood loss owing to religious or practical reasons.
Hyperbaric oxygen therapy can compensate for haemoglobin deficiency by
increasing the amount of dissolved oxygen in the plasma to a level
sufficient to maintain tissue oxygen delivery, even in the total absence
of red blood cells. Prolonged, continuous HBOT cannot, however, be used to
maintain life for the multiple days necessary for autologous replacement
of red blood cells, as pulmonary oxygen toxicity becomes an intolerable
and eventually fatal side-effect. Literature reports suggest the potential
to use intermittent HBOT as a short-term measure to relieve hypoxic
symptoms in patients with otherwise intolerably low haemoglobin levels
while waiting for red blood cells to regenerate or in patients with
limited compatible donor options while waiting for compatible blood
products to be delivered.57 58 59 60
Past and future development of a hyperbaric oxygen
therapy service in Hong Kong
The year 1994 witnessed a major development of HBOT
in Hong Kong. Previously, HBOT was provided by the British Royal Navy at
the HMS Tamar base and was confined to diving-related conditions. The
Recompression Treatment Centre was commissioned in 1994 by the Hong Kong
Government on Stonecutters Island and has become the major HBOT provider
since then. The facility, comprising a three-compartment multiplace
chamber, is managed by the Fire Services Department under the medical
supervision of the Occupational Medicine Division of the Department of
Health. Nonetheless, it is primarily used during diver training by the
Fire Services Department. Medical use is mainly for emergency DCI
treatment and CO poisoning cases referred from public hospitals, and many
elective sessions are devoted to radionecrosis treatment.61 Its remote location and lack of back-up critical care
facilities, however, render it unsuitable for critically ill patients
because of the risks inherent in patient transportation. Furthermore, lack
of properly trained local hyperbaric physicians and expertise as well as a
lack of human resources and training opportunities hinder the development
of HBOT in Hong Kong. Low awareness among physicians and patients makes
the referral for this treatment even less frequent. Although HBOT is also
provided by a private centre in Hong Kong, patient access remains very
limited.
One of the most important questions to address in
developing an HBOT service in Hong Kong is its service need in our
locality. Current data from the Recompression Treatment Centre (200-300
sessions for 20-30 patients per year) offer limited insight since access
to the service is limited. To assess the need accurately, it would be
necessary to estimate the number of patients in Hong Kong in whom HBOT is
indicated and the proportion of persons with each condition who might
benefit from HBOT. Doing so would depend on multiple factors including
considering alternative treatments available for each condition, cost and
financing, and doctor and patient beliefs and acceptance. Unfortunately,
treatment thresholds for each of the many possible indications are not
widely agreed on, or even researched, and the appropriate criteria for
referral vary widely in practice, between nations and even between
locations within nations. It must also be acknowledged that the referral
rates for HBOT are strongly influenced by its availability, integration
into the health care system, and the reputation of individual facilities
and their clinical leaders.
One way of estimating the need for HBOT service is
to study the data at health system level on the number of chambers and
activity level in other countries. Internationally, Australia offers a
good example. There, the eight major HBOT facilities are evenly
distributed, with one major government hospital-based facility in each of
its seven states and territory capital cities, excluding Canberra, plus
one in Townsville, the major infrastructure city serving North Queensland
and the Great Barrier Reef tourism zone. In addition, there are four
active private facilities and several more being planned. Each of the
major hospital-based facilities operates a large multiplace chamber, most
commonly of ‘triple lock’ (three compartment) design, that can be
configured to provide critical care as well as ambulatory care. Most
facilities also operate one or more monoplace chambers to provide
flexibility and allow efficient staff utilisation.
All government hospital-based facilities in
Australia are integrated with major academic tertiary hospitals. The
indications and thresholds for HBOT in Australia are conservative by
international standards and can therefore be seen as a good guide to what
would be a reasonable aim for Hong Kong. With 12 facilities serving a
population of approximately 24 million in Australia, each facility
provides 2000 to 5000 treatment sessions every year. While emergency
patients receive one to several treatment sessions, non-emergency patients
typically receive 20 to 40 treatment sessions spanning 4 to 8 weeks. The
workload is in the range of 2000 to 5000 HBOT sessions for 100 to 350
patients per centre per year, with two to three scheduled 2-h sessions per
day and six to ten non-emergency patients per scheduled session.
Hong Kong has a population of 7 million and there
exists a need for at least one HBOT hospital-based facility, especially
for critically ill patients. In 2010, a task force was set up in the
Hospital Authority to review the development of HBOT. Approval was finally
given in 2014 to establish the first hospital-based HBOT centre at the
Pamela Youde Nethersole Eastern Hospital. This new centre will be managed
by the Accident and Emergency Department in collaboration with the
Intensive Care Unit. The facility has been designed to be close to the
resuscitation room of the Accident and Emergency Department and the
service will be operationally supported by the Intensive Care Unit. With
the establishment of the first public hospital-based HBOT, it is expected
that it will be possible to offer additional treatment options for
conditions where HBOT is indicated. For instance, DCI and CO-poisoned
patients can be referred to the Pamela Youde Nethersole Eastern Hospital
for screening for suitability for HBOT and management. Life-threatening
infections, such as gas gangrene and NSTI, and limb-threatening crush
injuries can be managed with HBOT as an adjunct to conventional therapy.
It is also expected that HBOT will be made available to many patients with
chronic conditions such as non-healing diabetic wounds, compromised skin
flaps and grafts, and radionecrosis in the out-patient setting. The
hospital-based HBOT centre will also provide more opportunity for local
training and research.
Conclusion
With the opening of the first hospital-based HBOT
centre in Hong Kong in 2018, management of conditions such as DCI, CO
poisoning, NSTI, and acute crush injuries may change dramatically, in
terms of treatment choices as well as the logistics of patient transfer
between hospitals. Involvement of emergency physicians as facilitators,
modulators, and coordinators in this treatment will also widen the scope
of HBOT application and strengthen collaboration with other disciplines.
Declaration
The authors have no conflicts of interest to
disclose.
References
1. Clarke D. History of hyperbaric therapy.
In: Neuman TS, Thom SR, editors. Physiology and Medicine of Hyperbaric
Oxygen. Philadelphia: Saunders; 2008: 3-23.
2. Weaver LK, editor. Hyperbaric Oxygen
Therapy Indications. 13th ed. North Palm Beach, FL: Best Publishing
Company; 2014.
3. Hardy K. The physics of hyperbaric
oxygen therapy. In: Neuman TS, Thom SR, editors. Physiology and medicine
of hyperbaric oxygen. Philadelphia: Saunders; 2008: 57-64.
4. Mathieu D. Contraindications to
hyperbaric oxygen therapy. In: Neuman TS, Thom SR, editors. Physiology and
medicine of hyperbaric oxygen. Philadelphia: Saunders; 2008: 587-98.
5. Fukaya E, Hopf HW. HBO and gas embolism.
Neurol Res 2007;29:142-5. Crossref
6. Muth CM, Shank ES. Gas embolism. N Engl
J Med 2000;342:476-82. Crossref
7. Gronert GA, Messick JM Jr, Cucchiara RF,
Michenfelder JD. Paradoxical air embolism from a patent foramen ovale.
Anesthesiology 1979;50:548-9. Crossref
8. Van Liew HD, Flynn ET. Direct ascent
from air and N2-O2 saturation dives in humans: DCS risk and evidence of a
threshold. Undersea Hyperb Med 2005;32:409-19.
9. Vann RD, Butler FK, Mitchell SJ, Moon
RE. Decompression illness. Lancet 2011;377:153-64. Crossref
10. Branger AB, Lambertsen CJ, Eckmann DM.
Cerebral gas embolism absorption during hyperbaric therapy: theory. J Appl
Physiol (1985) 2001;90:593-600. Crossref
11. Trytko BE, Bennett MH. Arterial gas
embolism: a review of cases at Prince of Wales Hospital, Sydney, 1996 to
2006. Anaesth Intensive Care 2008;36:60-4.
12. Moon RE, Gorman DF. Treatment of the
decompression disorders. In: Brubakk AO, Neuman TS, editors. Bennett and
Elliot’s Physiology and Medicine of Diving. 5th ed. London: WB. Saunders
Company Ltd; 2003: 600-50.
13. Moon RE, Sheffield PJ. Guidelines for
treatment of decompression illness. Aviat Space Environ Med
1997;68:234-43.
14. Thom SR. Antidote in depth: hyperbaric
oxygen. In: Hoffman RS, Howland MA, Lewin NA, Nelson LS, Goldfrank LR,
editors. Goldfrank’s Toxicologic Emergencies. 10th ed. New York: McGraw
Hill Education; 2015: 1594-601.
15. Sircar K, Clower J, Shin MK, Bailey C,
King M, Yip F. Carbon monoxide poisoning deaths in the United States, 1999
to 2012. Am J Emerg Med 2015;33:1140-5. Crossref
16. Weaver LK. Clinical practice. Carbon
monoxide poisoning. N Engl J Med 2009;360:1217-25. Crossref
17. Roderique JD, Josef CS, Feldman MJ,
Spiess BD. A modern literature review of carbon monoxide poisoning
theories, therapies, and potential targets for therapy advancement.
Toxicology 2015;334:45-58.
18. Hampson NB, Piantadosi CA, Thom SR,
Weaver LK. Practice recommendations in the diagnosis, management, and
prevention of carbon monoxide poisoning. Am J Resp Crit Care
2012;186:1095-101. Crossref
19. Thom SR, Fisher D, Zhang J, Bhopale
VM, Cameron B, Buerk DG. Neuronal nitric oxide synthase and
N-methyl-D-aspartate neurons in experimental carbon monoxide poisoning.
Toxicol Appl Pharmacol 2004;194:280-95.
20. Peterson JE, Stewart RD. Absorption
and elimination of carbon monoxide by inactive young men. Arch Environ
Health 1970;21:165-71. Crossref
21. Brown SD, Piantadosi CA. Recovery of
energy metabolism in rat brain after carbon monoxide hypoxia. J Clin
Invest 1992;89:666-72. Crossref
22. Thom SR. Antagonism of carbon
monoxide-mediated brain lipid peroxidation by hyperbaric oxygen. Toxicol
Appl Pharmacol 1990;105:340-4. Crossref
23. Thom SR. Functional inhibition of
leukocyte β2 integrins by hyperbaric oxygen in carbon monoxide-mediated
brain injury in rats. Toxicol Appl Pharmacol 1993;123:248-56. Crossref
24. Thom SR, Mendiguren I, Hardy K, et al.
Inhibition of human neutrophil β2-integrin-dependent adherence by
hyperbaric O2. Am J Physiol 1997;272(3 Pt 1):C770-7. Crossref
25. Thom SR, Bhopale VM, Fisher D.
Hyperbaric oxygen reduces delayed immune-mediated neuropathology in
experimental carbon monoxide toxicity. Toxicol Appl Pharmacol
2006;213:152-9. Crossref
26. Smith G, Sharp GR. Treatment of
carbon-monoxide poisoning with oxygen under pressure. Lancet
1960;276:905-6. Crossref
27. Raphael JC, Elkharrat D,
Jars-Guincestre MC, et al. Trial of normobaric and hyperbaric oxygen for
acute carbon monoxide intoxication. Lancet 1989;2:414-9. Crossref
28. Thom SR, Taber RL, Mediguren II, Clark
JM, Hardy KR, Fisher AB. Delayed neuropsychologic sequelae after carbon
monoxide poisoning: prevention by treatment with hyperbaric oxygen. Ann
Emerg Med 1995;25:474-80. Crossref
29. Mathieu D, Wattel F, Mathieu-Nolf M,
et al. Randomized prospective study comparing the effect of HBO versus 12
hours NBO in non comatose CO poisoned patients: results of the interim
analysis [abstract]. Undersea Hyperb Med 1996;23:7-8.
30. Scheinkestel CD, Bailey M, Myles PS,
et al. Hyperbaric or normobaric oxygen for acute carbon monoxide
poisoning: a randomised controlled clinical trial. Med J Aust
1999;170:203-10.
31. Weaver LK, Hopkins RO, Chan KJ, et al.
Hyperbaric oxygen for acute carbon monoxide poisoning. N Engl J Med
2002;347:1057-67. Crossref
32. Annane D, Chadda K, Gajdos P,
Jars-Guincestre MC, Chevret S, Raphael JC. Hyperbaric oxygen for acute
domestic carbon monoxide poisoning: two randomized controlled trials.
Intensive Care Med 2011;37:486-92. Crossref
33. Buckley NA, Juurlink DN, Isbister G,
Bennett MH, Lavonas EJ. Hyperbaric oxygen for carbon monoxide poisoning.
Cochrane Database Syst Rev 2011;(4):CD002041. Crossref
34. American College of Emergency
Physicians Clinical Policies Subcommittee (Writing Committee) on Carbon
Monoxide Poisoning; Wolf SJ, Maloney GE, Shih RD, Shy BD, Brown MD.
Clinical policy: Critical issues in the evaluation and management of adult
patients presenting to the emergency department with acute carbon monoxide
poisoning. Ann Emerg Med 2017;69:98-107.e6. Crossref
35. Huang CC, Ho CH, Chen YC, et al.
Hyperbaric oxygen therapy is associated with lower short- and long-term
mortality in patients with carbon monoxide poisoning. Chest
2017;152:943-53. Crossref
36. Mutluoglu M, Metin S, Ibrahim Arziman,
Uzun G, Yildiz S. The use of hyperbaric oxygen therapy for carbon monoxide
poisoning in Europe. Undersea Hyperb Med 2016;43:49-56.
37. Van Hoesen KB, Camporesi EM, Moon RE,
Hage ML, Piantadosi CA. Should hyperbaric oxygen be used to treat the
pregnant patient for acute carbon monoxide poisoning? A case report and
literature review. JAMA 1989;261:1039-43. Crossref
38. Elkharrat D, Raphael JC, Korach JM, et
al. Acute carbon monoxide intoxication and hyperbaric oxygen in pregnancy.
Intensive Care Med 1991;17:289-92. Crossref
39. Yarar C, Yahut A, Akin A, Yildiz B,
Dinleyici EC. Analysis of the features of acute carbon monoxide poisoning
and hyperbaric oxygen therapy in children. Turk J Pediatr 2008;50:235-41.
40. Waisman D, Shupak A, Weisz G, Melamed
Y. Hyperbaric oxygen therapy in the pediatric patient: the experience of
the Israel Naval Medical Institute. Pediatrics 1998;102:E53. Crossref
41. Liebelt EL. Hyperbaric oxygen therapy
in childhood carbon monoxide poisoning. Curr Opin Pediatr 1999;11:259-64.
Crossref
42. Cimşit M, Uzun G, Yildiz S. Hyperbaric
oxygen therapy as an anti-infective agent. Expert Rev Anti Infect Ther
2009;7:1015-26. Crossref
43. Kaye D. Effect of hyperbaric oxygen on
Clostridia in vitro and in vivo. Proc Soc Exp Biol Med
1967;124:360-6. Crossref
44. Unnika V. Inhibition of toxin
production in Clostridium perfringens in vitro by hyperbaric
oxygen. Antonie Van Leeuwenhoek 1965;31:181-6. Crossref
45. Jacoby I. Clostridial myositis,
necrotizing fasciitis, and zygomycotic infections. In: Neuman TS, Thom SR,
editors. Physiology and medicine of hyperbaric oxygen therapy.
Philadelphia: Saunders Elsevier; 2008: 397-418.
46. Wilkinson D, Doolette D. Hyperbaric
oxygen treatment and survival from necrotizing soft tissue infection. Arch
Surg 2004;139:1339-45. Crossref
47. Escobar SJ, Slade JB Jr, Hunt TK,
Cianci P. Adjuvant hyperbaric oxygen therapy (HBO2) for treatment of
necrotizing fasciitis reduces mortality and amputation rate. Undersea
Hyperb Med 2006;32:437-43.
48. Devaney B, Frawley G, Frawley L,
Pilcher DV. Necrotizing soft tissue infections: the effect of hyperbaric
oxygen on mortality. Anaesth Intensive Care 2015;43:685-92.
49. Shaw JJ, Psoinos C, Emhoff TA, Shah
SA, Santry HP. Not just full of hot air: hyperbaric oxygen therapy
increases survival in cases of necrotizing soft tissue infections. Surg
Infect (Larchmt) 2014;15:328-35. Crossref
50. Brown DR, Davis NL, Lepawsky M,
Cunningham J, Kortbeek J. A multicenter review of the treatment of major
truncal necrotizing infections with and without hyperbaric oxygen therapy.
Am J Surg 1994;167:485-9. Crossref
51. Shupak A, Shoshani O, Goldenberg I,
Barzilai A, Moskuna R, Bursztein S. Necrotizing fasciitis: an indication
for hyperbaric oxygenation therapy? Surgery 1995;118:873-8. Crossref
52. Levett D, Bennett MH, Millar I.
Adjunctive hyperbaric oxygen for necrotizing fasciitis. Cochrane Database
Syst Rev 2015;(1):CD007937. Crossref
53. Strauss MB. The effect of hyperbaric
oxygen in crush injuries and skeletal muscle-compartment syndromes.
Undersea Hyperb Med 2012;39:847-55.
54. Bouachour G, Cronier P, Gouello JP,
Toulemonde JL, Talha A, Alquier P. Hyperbaric oxygen therapy in the
management of crush injuries: a randomized double-blind placebo-controlled
clinical trial. J Trauma 1996;41:333-9. Crossref
55. Garcis-Covarrubias L, McSwain NE Jr,
Van Meter K, Bell RM. Adjuvant hyperbaric oxygen therapy in the management
of crush injury and traumatic ischemia: an evidence-based approach. Am
Surg 2005;71:144-51.
56. Millar IL, McGinnes RA, Williamson O,
et al. Hyperbaric Oxygen in Lower Limb Trauma (HOLLT); protocol for a
randomised controlled trial. BMJ Open 2015;5:e008381. Crossref
57. Hart GB. Exceptional blood loss
anemia. Treatment with hyperbaric oxygen. JAMA 1974;228:1028-9. Crossref
58. McLoughlin PL, Cope TM, Harrison JC.
Hyperbaric oxygen therapy in the management of severe acute anaemia in a
Jehovah’s witness. Anaesthesia 1999;54:891-5. Crossref
59. Greensmith JE. Hyperbaric oxygen
reverses organ dysfunction in severe anemia. Anesthesiology
2000;93:1149-52. Crossref
60. Graffeo C, Dishong W. Severe blood
loss anemia in a Jehovah’s Witness treated with adjunctive hyperbaric
oxygen therapy. Am J Emerg Med 2013;31:756.e3-4. Crossref
61. Ramaswami RA, Lo WK. Use of hyperbaric
oxygen therapy in Hong Kong. Hong Kong Med J 2000;6:108-12.