Hong Kong Med J 2017 Apr;23(2):168–76 | Epub 17 Mar 2017
DOI: 10.12809/hkmj166070
© Hong Kong Academy of Medicine. CC BY-NC-ND 4.0
REVIEW ARTICLE
Clinical use of venovenous extracorporeal membrane oxygenation
George WY Ng, FCICM, FHKAM (Medicine);
Henry J Yuen, FHKCA(Intensive Care), FHKAM (Anaesthesiology);
KC Sin, FHKCP, FHKAM (Medicine);
Anne KH Leung, FCICM, FHKAM (Anaesthesiology);
KW Au Yeung, FCICM, FHKAM (Anaesthesiology);
KY Lai, FRCP (Edin), FHKAM (Medicine)
Department of Intensive Care, Queen Elizabeth Hospital, Jordan, Hong
Kong
Corresponding author: Dr George WY Ng (georgeng77@yahoo.com)

Abstract
Extracorporeal membrane oxygenation has been
used clinically for more than 40 years. The technique
provides respiratory and/or circulatory support
via venovenous and veno-arterial configurations,
respectively. We review the basic physiological
principles of extracorporeal membrane oxygenation
systems in venovenous extracorporeal membrane
oxygenation. Clinical aspects including patient
selection, equipment, setup, and specific patient
management are outlined. Pros and cons of the
use of extracorporeal membrane oxygenation in
respiratory failure are discussed.
Introduction
Extracorporeal membrane oxygenation (ECMO)
provides temporary extracorporeal support of the
respiratory and/or cardiac system for patients who
fail to respond to conventional therapies, buying
time for recovery from the underlying condition,
and for specific treatment to take effect. The
concept and technology of ECMO originated from
cardiopulmonary bypass that was first used in 1953.1
The first case of successful ECMO support in an
adult patient was published by Hill et al in 1972.2 3 Currently, ECMO use is not confined to the operating
theatre, but has been extended to the intensive care
unit and during inter-hospital transfer.
According to the Extracorporeal Life Support
Organization (ELSO) Registry report, 86 287 patients
received extracorporeal life support (ECLS) globally
(up to January 2017).4 The majority of patients were
neonates (44.8%), while 24.1% were children and
31.1% were adults. The types of ECLS included
respiratory support (58.4%), cardiac support (31.9%),
and extracorporeal cardiopulmonary resuscitation
(9.7%).4
Types of extracorporeal membrane oxygenation
There are two main types of ECMO configuration:
venovenous (VV) and veno-arterial (VA)—VV-ECMO provides solely lung support, while
VA-ECMO provides both heart and lung support.
Depending on the clinical indications and disease
progress, the ECMO circuit can be interchanged
between VV and VA, from VV to high-flow VV or
from VV to veno-arterial-venous ECMO.
Configuration of extracorporeal membrane oxygenation
The ECMO circuit consists of an access cannula that
drains deoxygenated venous blood from the patient,
a pump, an artificial lung (oxygenator) that provides
oxygenation and carbon dioxide (CO2) removal, a
heat exchanger, and a return cannula that returns
oxygenated blood via a central vein (ie VV-ECMO)
or major artery (ie VA-ECMO).
Venovenous extracorporeal membrane oxygenation
The technique VV-ECMO provides only lung
support (Fig 1a). The circuit is connected in series
with the right heart and the lungs with the access
cannula commonly inserted at the femoral vein.
Deoxygenated blood is drained from the venous
circulation by a motor pump. The deoxygenated
blood then passes through an oxygenator where
oxygen diffuses across the oxygenator membrane
into the blood. The oxygenated blood then returns
to the right heart via a return cannula, placed at the
femoral vein or the right internal jugular vein.
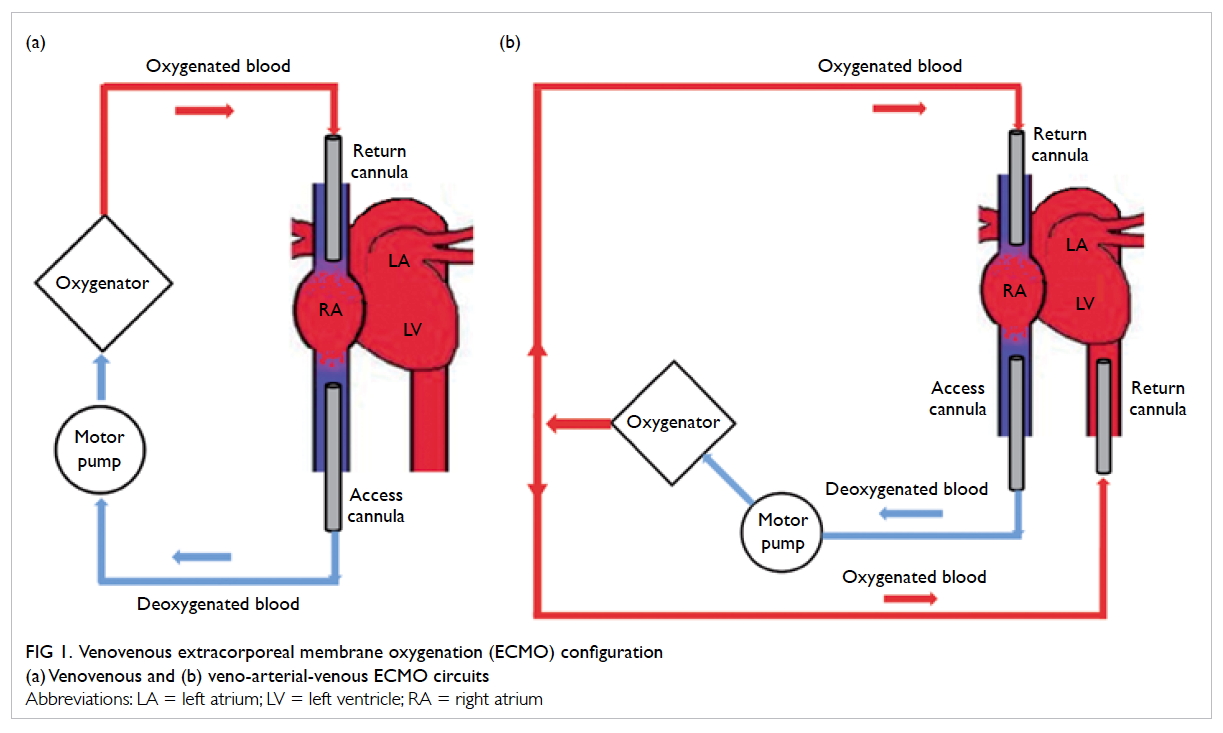
Figure 1. Venovenous extracorporeal membrane oxygenation (ECMO) configuration
(a) Venovenous and (b) veno-arterial-venous ECMO circuits
High-flow venovenous extracorporeal membrane oxygenation
The maximal blood flow that can be achieved in an
ECMO system is determined by elements of the
Hagen-Poiseuille equation, of which the key factor is
the cannula size. In situations where maximal blood
flow is unable to meet the metabolic demand of the
patient, such as sepsis with high cardiac output or large
body size, an additional access cannula is inserted.
Veno-arterial-venous extracorporeal membrane oxygenation
Patients with acute severe respiratory failure
sometimes develop refractory shock due to severe
sepsis and/or myocardial ischaemia. In this case,
veno-arterial-venous ECMO can provide both
respiratory and circulatory support (Fig 1b). This
is achieved by the insertion of an additional return
cannula into a major artery in the existing VV-ECMO
system, such that a proportion of the oxygenated
blood is diverted to the arterial circulation to support
organ perfusion.
Physiology of the extracorporeal membrane oxygenation system
In the native lungs, gaseous exchange occurs by
diffusion at the blood gas interface in the alveoli. In
the ECMO oxygenator, the same principle applies.
The efficiency of gaseous exchange depends on the
driving force of the gas and the diffusion efficiency.
The driving force of a gas is related to the partial
pressure difference across the blood gas interface for
the individual gas. The efficiency of diffusion depends
on the area for diffusion, distance over which gas has
to pass, and the diffusion coefficient for the individual
gas. The amount of oxygen delivered by VV-ECMO
is influenced by the oxygenator membrane surface
area, membrane thickness, haemoglobin level, and
ECMO blood flow.
The venous partial pressure of oxygen (PaO2)
at the blood interface of the oxygenator is around 5.3
kPa, while dry 100% oxygen (O2) runs through the gas
interface equivalent to a partial pressure of 101 kPa.
This provides a partial pressure gradient for oxygen
to diffuse across the semipermeable membrane of
the oxygenator from the gas interface to the blood
interface.5 An example is shown in the Box.
In reality, the maximal oxygen transfer
capability is also determined by the gas exchange
surface area and the amount of disruption to laminar
flow as blood passes through the oxygenator.
The O2 consumption (VO2) is approximately
250 mL/min in a VV-ECMO adult patient.6 Thus from the above example, the DO2/VO2 ratio is greater than 2:1 and within the safe range to prevent anaerobic metabolism (DO2 = oxygen
delivery). If the ECMO blood flow is 4 L/min and the
cardiac output is 5 L/min, 1 L of blood that returns
to the right heart theoretically passes through the
pulmonary circulation and the native sick lungs
achieving limited gaseous exchange. Therefore,
the final O2 saturation will not be 100% due to this
admixture.
Active sepsis results in both increased VO2 and increased cardiac output. In
most situations, the native sick lungs can still
partially serve the function of gaseous exchange
and contribute to DO2. Nonetheless when
DO2/VO2 ratio falls below 2:1, anaerobic respiration
occurs with consequent accumulation of lactate.
Similar to O2, CO2 can pass through the
oxygenator membrane from the blood to the gas
interface by diffusion; CO2 has a higher diffusion
coefficient than O2 because of its greater blood
solubility. As a result, CO2 transfer is 6 times
faster than O2 for the same membrane thickness
and pressure gradient. Unlike O2, CO2 transfer is
independent of blood flow. The most important
limiting factor for CO2 transfer is the relative
concentration of CO2 on either side of the membrane.
The partial pressure of CO2 in the blood interface is
about 6 kPa, but there is negligible CO2 in the gas
interface of the membrane. It is possible to remove
250 mL/min of CO2 with an ECMO blood flow of
<1 L/min by flushing the membrane lung with fresh
gas flow (Sweep gas) to maintain the high CO2
gradient across the membrane.
Venovenous extracorporeal membrane oxygenation: patient
selection and clinical evidence
Mechanical ventilation is the mainstay treatment
for respiratory failure in patients with severe acute
respiratory distress syndrome (ARDS). The use of
positive pressure ventilation, however, can induce
lung injury by over-distension of the alveoli or from
cyclical collapsing and re-opening of the alveoli.
Moreover, ventilation-induced lung injury can
trigger a systemic inflammatory response and result
in multi-organ failure.7
A lung protective ventilation strategy is proven
to reduce ventilator-associated lung injury and
mortality in ARDS patients.7 Yet in clinical practice,
it is often difficult to adhere to these ventilator
parameters without causing significant hypercarbia
and respiratory acidosis. Of note, ECMO can
overcome this challenge as it provides an additional
source of blood oxygenation and CO2 removal
through the extracorporeal circuit and oxygenator,
and allows lung rest. Table 18 lists the common
indications for and contra-indications to VV-ECMO.
Careful patient selection for VV-ECMO is
crucial to achieve a favourable outcome, balancing
the perceived benefit of improving oxygenation
with the risk of complications during ECMO
initiation and maintenance. Alternatives to ECMO
including prone ventilation and inhaled nitric oxide
are also considered in this risk-benefit assessment.
Indeed, VV-ECMO for severe ARDS is currently
established as a sound rescue therapy rather than
a standard of care. The landmark VV-ECMO trial
(CESAR: Conventional ventilatory support versus
Extracorporeal membrane oxygenation for Severe
Adult Respiratory failure) was the first to report
improved survival in patients with severe ARDS
transferred to an ECMO referral centre compared
with those who received conventional treatment.9
This—together with good outcomes from
Australasian,10 French,11 and Italian12 experiences
in patients with H1N1-associated ARDS—has
contributed to the heightened interest in VV-ECMO
and its increased use during and after the H1N1
pandemic.
The results of the CESAR trial could be
interpreted as a recommendation for transfer to
an ECMO-capable centre, where the improved
outcomes may have been from the total care at the
ECMO centre, and not from ECMO therapy alone.9
Nonetheless no randomised controlled trial has
proven that VV-ECMO should be the standard of
care for severe ARDS in non-influenza patients. The
ongoing ECMO for severe ARDS (EOLIA) trial is a
multicentre, international, randomised controlled
trial evaluating early ECMO (within 3-6 hours) after
the diagnosis of very severe ARDS (PaO2/FiO2 <80
mm Hg, where FiO2 = fraction of inspired oxygen)
in patients not responding favourably to optimal
ventilation. A positive outcome from this trial may
further support the use of VV-ECMO for respiratory
failure, and prompt a review of our territory-wide
referral network for coordinated transfers to improve
patient care.13
An ELSO Centre of Excellence recently reported
the long-term outcome for patients with respiratory
failure or sepsis treated with ECMO from 1995 to
2013 with 64% survival to discharge and better long-term
survival for patients treated for infection.14 This
suggests that the reversibility of the disease process
is an important consideration when selecting
appropriate patients for ECMO.
Different ECMO centres have proposed their
own selection criteria for ECMO treatment.15 16 A pretreatment predicting a mortality of higher than
80% is widely accepted as a cut-off for screening a
potential ECMO candidate. In addition, ELSO
suggests that this 80% mortality risk broadly
corresponds to a PaO2/FiO2 of <100 mm Hg on FiO2
>90% and/or Murray score of 3-4 despite optimal care
for ≥6 hours.8 In addition, the longer the duration
of mechanical ventilation before initiation of ECMO,
the worse the outcome.17 It was also reported that
ECMO patients had a better survival rate when they
were managed in an experienced quaternary referral
ECMO centre.9
Murray score
In 1988, Murray et al18 proposed an extended
definition of ARDS, taking into account various
pathophysiological features of the clinical
syndrome. The Murray scoring system includes
four components for stratification of the severity
of ARDS. Each component is scored from 0 to 4
according to severity (Table 218). The final mean
score is obtained by dividing the collective score
by four, which is the number of components used.
A score of 0 indicates no lung injury, 1-2.5 indicates
mild-to-moderate lung injury, and >2.5 indicates the
presence of ARDS.
Equipment, setup, and specific patient management
Equipment
As an ECLS system, there are three key components
of an ECMO circuit—blood pump, oxygenator, and
cannula.
The function of the blood pump is to provide
the required blood flow to the patient. This can be
achieved by means of mechanical (roller pump) or
centrifugal force (centrifugal pump). With advances
in technology, a centrifugal pump has replaced the
roller pump in ECMO as it is more durable, lighter,
and results in less haemolysis, and less platelet
and complement activation. A centrifugal pump is
preload sensitive and afterload dependent. ‘Preload
sensitive’ means the blood flow is sensitive to volume
at the pump inlet. In the presence of inadequate
blood drainage such as in hypovolaemia, the flow
reduces automatically. ‘Afterload dependent’ means
the blood flow is also sensitive to resistance at the
pump outlet. Flow reduction occurs when post-pump
resistance increases. Examples include the
presence of thrombus in the oxygenator, kinking of
the return cannula, and excessive systemic vascular
resistance or mean arterial pressure.
The oxygenator was traditionally made
of silicone membrane because of their high
biocompatibility. It was later replaced by
microporous polypropylene hollow fibres with an
advantage of lower pressure drop, need for lower
priming volume, and better gas exchange efficiency.
Direct contact between the gas interface and blood
through the microporous fibres, however, results
in plasma leakage, which is a serious complication.
The newest-generation oxygenator contains multiple
hollow fibres that are coated with polymethylpentene
and avoids direct contact between the gas interface
and blood. Gas transfer is by diffusion. This new
oxygenator is more efficient (with lower volume),
more effective in gas exchange, causes less platelet
and plasma protein loss, and has a thrombo-resistant
coating.19
An ideal ECMO cannula provides the desired
flow with minimal pressure drop, and minimal
damage to blood cells or activation of inflammatory
response. According to the Hagen-Poiseuille
equation, a cannula with the shortest length and the
largest diameter offers the greatest flow. Blood in
contact with the inner layer of a cannula activates
the coagulation and complement cascades, the
kallikrein-kinin system, and other inflammatory
cells. Currently available cannulas have a special
surface coating to minimise the risk of thrombus
formation. An example of such coating is a heparin-coated
surface although there remains a risk of
developing heparin-induced thrombocytopenia.
Newer technology employs a hydrophilic layer
with negatively charged groups to repel negatively
charged inflammatory proteins and platelets.20
Setup
Role prioritisation and good communication are
the keys for successful ECMO initiation and setup.
Informed consent should be obtained from the
patient’s family. The patient should be well-sedated,
and coagulopathy (if any) should be corrected before
cannulation.
The cannulation procedure is usually
performed at the bedside under aseptic conditions.
The Seldinger technique is employed with serial
dilatation under ultrasound or fluoroscopic
guidance. For VV-ECMO, one common approach is
a two-cannula technique with either femoral-jugular
or femoral-femoral cannulation. Some centres use
a single cannula technique and insert a dual lumen
catheter (Avalon Laboratories, Rancho Dominguez
[CA], United States) into the right internal jugular vein. If
a femoral-jugular approach for the two-cannula
technique is used, the patient should be placed in
the Trendelenburg position. The left internal jugular
vein is a less preferred site due to its smaller size
and higher risk of vascular injury at the internal
jugular-brachiocephalic vein junction. Ultrasound
is commonly used during the cannulation procedure
to locate the vessel, assess vessel size, and to confirm
correct guidewire placement and final position of the
cannula. Systemic anticoagulation should be started
immediately after cannulation provided there is no
absolute contra-indication.
Specific patient management
Ventilation strategies
Patients with severe ARDS often have both
hypoxaemia and hypercarbia that make the setting
of ventilator parameters difficult. Inappropriate
setting of mechanical ventilation can lead to further
lung damage including excessive transpulmonary
pressure (barotrauma), excessive lung volume
inside the alveoli (volutrauma), and shearing
stress during repetitive opening and closing of the
alveoli (atelectrauma).21 Several large randomised
controlled trials have proven that a low tidal volume
strategy (≤6 mL/kg of ideal body weight) achieves
better outcomes than a traditional ventilatory
strategy (10-12 mL/kg of ideal body weight ).7 22 23 In
addition, maintaining a plateau pressure of <30 cm
H2O minimises the risk of barotrauma. It is hard to
achieve these targets in a sick lung using mechanical
ventilation alone.
The use of ECMO overcomes the problem as
CO2 removal and blood oxygenation take place in
an extracorporeal system. This permits complete
lung rest with the application of low tidal volume
and plateau pressure (ie lung protective ventilation
strategy).24 One of the ventilation strategies
recommended by ELSO guidelines is as follows8:
pressure-controlled ventilation at positive end-expiratory pressure (PEEP) 15 cm H2O and pressure
control above PEEP at 10 cm H2O, rate 5/min, inspiratory-to-expiratory time ratio 2:1, and FiO2 50%.
The optimal ventilator strategy for patients
on VV-ECMO, especially the level of PEEP, is still
controversial. Randomised controlled trials show that
in patients where a higher PEEP (14-15 cm H2O)
was used, there were fewer mechanical ventilator
days and number of organ failure compared with
patients using a lower PEEP (9-10 cm H2O). There
was, however, no mortality benefit. A criticism of
the randomised controlled trials is that they recruited
a large proportion of patients in whom PEEP was
inappropriate and who consequently did not respond to its application.25 26 27 More importantly, all these
studies were performed in non-ECMO settings.
A conservative fluid strategy is commonly
adopted in patients on VV-ECMO for ARDS and
may be associated with lower mortality, better
lung function, and shorter duration of mechanical
ventilation.28 Nonetheless, volume deficit can lead
to ECMO blood flow reduction as the centrifugal
pump is preload sensitive. Line shaking can occur if
volume depletion has become significant with pump
flow reduction.
Renal replacement therapy
Previous studies showed that 70% of ECMO patients
who developed acute kidney injury had a poorer
outcome.29 30 31 Continuous renal replacement therapy
(CRRT) is commonly used in ECMO patients for
renal support and fluid management. There are
various methods for delivering CRRT in ECMO
patients: by inserting a new venous cannula for
CRRT independent of the ECMO circuit, integrating
the haemofiltration filter into the ECMO circuit, or
by connecting a CRRT machine to the ECMO circuit
(Fig 2). To minimise the risk of air embolism, the CRRT circuit should be connected after the ECMO
motor pump.32 33 This combination enables a longer filter life and more accurate fluid management.34
Sedation
There are special concerns regarding the choice of
sedative agents in ECMO patients. The ECMO circuit
tubing absorbs lipophilic drugs. As such, lipophilic
sedative agents such as propofol, midazolam, and
fentanyl are rapidly removed from the systemic
circulation and larger doses are required to achieve
the target effect.35 36 Medications that are formulated as lipid suspensions, such as propofol, can also
corrode the oxygenator membrane, especially when
there is a high infusion rate.37 These agents should
be avoided.
Anticoagulation
Haemorrhagic and thromboembolic events are
common and are the main causes of morbidity and mortality in ECMO patients. Anticoagulation
is necessary during ECMO because of the high
thrombogenicity associated with blood contact
with a non-biological surface.38 Close monitoring is
nonetheless essential to avoid overanticoagulation.
Activated clotting time is frequently used
as a point-of-care monitoring of heparin in ECMO
patients,39 while activated partial thromboplastin
time is a conventional method for testing
the intrinsic and common coagulation pathways.
Unfractionated heparin is widely used as the
anticoagulant for ECMO patients although potential
complications including bleeding, heparin-induced
thrombocytopenia, and tachyphylaxis, may arise.40
Bivalirudin, a direct thrombin inhibitor, has been
tested in ECMO patients as an alternative to
heparin41 and been shown to cause less bleeding, be
more cost-effective, and results in the need for fewer
allogenic transfusions.42
Possible complications related to venovenous extracorporeal membrane oxygenation
Extracorporeal membrane oxygenation is a complex
and high-risk procedure. Medical crises occur rarely
but can be life-threatening (Table 3).4 Making the
correct decision hinges on a rapid understanding the
situation and the primary cause of the complication.
Weaning from extracorporeal membrane oxygenation
As the native lung improves, the demand on
the extracorporeal system decreases. In general,
ECMO weaning can be considered if the patient
has substantial signs of native lung recovery, and
can tolerate low ECMO support (ie ECMO blood
flow <2.0 L/min and lower sweep gas FiO2 to keep
peripheral oxygen saturation >95%).8 Decreasing the ECMO blood flow to <2.0 L/min, however, risks thrombus formation.
As an alternative, a weaning trial can be done by
either switching off the sweep gas flow or decreasing
the FiO2 to 0.21. The setting of the mechanical
ventilator is adjusted accordingly. Decannulation
can be considered if the patient is stable for >1 hour
following the adjustment.43
The ECMO cannulae are removed after heparin
infusion has been stopped for more than 60 minutes,
and there is no major coagulopathy. Cannulae that
were inserted by a cutdown procedure should be
removed in the operating theatre as vessel repair is
necessary. Those that were inserted percutaneously
can be removed at the bedside.
Conclusion
Venovenous ECMO is considered one of the
rescue treatments in patients who fail to respond
to conventional therapies. With the accumulation
of experience and advances in technology, the
application of VV-ECMO has been extended from
viral or bacterial pneumonia to other causes of
respiratory failure. The number of adult patients
receiving this therapy substantially increased
following the CESAR trial. One key principle
of patient selection is the underlying disease
reversibility. The VV-ECMO procedure should be
regarded as temporary lung support that serves
as a bridge to disease recovery or transplantation.
Individual ECMO centres may develop their own
inclusion and exclusion criteria based on availability
of expertise, resources, and supporting services.
Of note, ECMO is not risk free. Understanding the
equipment physiology, configuration, and potential
complications is essential for its safe application.
Meticulous monitoring combined with appropriate
patient management is crucial to the success of
VV-ECMO in ARDS patients.
References
1. Gibbon JH Jr, Hill JD. Part I. The development of the
first successful heart-lung machine. Ann Thorac Surg
1982;34:337-41. Crossref
2. Hill JD, O’Brien TG, Murray JJ, et al. Prolonged
extracorporeal oxygenation for acute post-traumatic
respiratory failure (shock-lung syndrome). Use of the
Bramson membrane lung. N Engl J Med 1972;286:629-34. Crossref
3. Hill JD, De Leval MR, Fallat RJ, et al. Acute respiratory
insufficiency. Treatment with prolonged extracorporeal
oxygenation. J Thorac Cardiovasc Surg 1972;64:551-62.
4. Extracorporeal Life Support Organization. ECLS registry
report, international summary 2017. Available from: https://www.elso.org/Registry/Statistics/InternationalSummary.aspx. Accessed Jan 2017.
5. Chauhan S, Subin S. Extracorporeal membrane
oxygenation, an anesthesiologist’s perspective: Physiology
and principles. Part 1. Ann Card Anaesth 2011;14:218-29. Crossref
6. Holzgraefe B, Broomé M, Kalzén H, Konrad D, Palmér
K, Frenckner B. Extracorporeal membrane oxygenation
for pandemic H1N1 2009 respiratory failure. Minerva
Anestesiol 2010;76:1043-51.
7. Ventilation with lower tidal volumes as compared with
traditional tidal volumes for acute lung injury and the acute
respiratory distress syndrome. The Acute Respiratory
Distress Syndrome Network. N Engl J Med 2000;342:1301-8. Crossref
8. ELSO adult respiratory failure supplement to the ELSO
general guidelines. Version 1.3. Ann Arbor, MI: Extracorporeal Life Support Organization; 2013.
9. Peek GJ, Mugford M, Tiruvoipati R, et al. Efficacy
and economic assessment of conventional ventilatory
support versus extracorporeal membrane oxygenation for
severe adult respiratory failure (CESAR): a multicentre
randomised controlled trial. Lancet 2009;374:1351-63. Crossref
10. Australia and New Zealand Extracorporeal Membrane
Oxygenation (ANZ ECMO) Influenza Investigators, Davies
A, Jones D, et al. Extracorporeal membrane oxygenation
for 2009 influenza A(H1N1) acute respiratory distress
syndrome. JAMA 2009;302:1888-95. Crossref
11. Pham T, Combes A, Rozé H, et al. Extracorporeal
membrane oxygenation for pandemic influenza A(H1N1)–induced acute respiratory distress syndrome: a cohort
study and propensity-matched analysis. Am J Respir Crit
Care Med 2013;187:276-85. Crossref
12. Patroniti N, Zangrillo A, Pappalardo F, et al. The Italian
ECMO network experience during the 2009 influenza
A(H1N1) pandemic: preparation for severe respiratory
emergency outbreaks. Intensive Care Med 2011;37:1447-57. Crossref
13. Extracorporeal membrane oxygenation for severe acute
respiratory distress syndrome (EOLIA). Available from:
https://clinicaltrials.gov/ct2/show/NCT01470703.
Accessed Dec 2016.
14. von Bahr V, Hultman J, Eksborg S, Frenckner B, Kalzén H.
Long-term survival in adults treated with extracorporeal
membrane oxygenation for respiratory failure and sepsis.
Crit Care Med 2017;45:164-70. Crossref
15. Lewandowski K. Extracorporeal membrane oxygenation
for severe acute respiratory failure. Crit Care 2000;4:156-68. Crossref
16. Vasilyev S, Schaap RN, Mortensen JD. Hospital survival
rates of patients with acute respiratory failure in modern
respiratory intensive care units. An international,
multicenter, prospective survey. Chest 1995;107:1083-8. Crossref
17. Pranikoff T, Hirschl RB, Steimle CN, Anderson HL
3rd, Bartlett RH. Mortality is directly related to the
duration of mechanical ventilation before the initiation of
extracorporeal life support for severe respiratory failure.
Crit Care Med 1997;25:28-32. Crossref
18. Murray JF, Matthay MA, Luce JM, Flick MR. An expanded
definition of the adult respiratory distress syndrome. Am
Rev Respir Dis 1988;138:720-3. Crossref
19. Formica F, Avalli L, Martino A, et al. Extracorporeal
membrane oxygenation with a poly-methylpentene
oxygenator (Quadrox D). The experience of a single Italian
centre in adult patients with refractory cardiogenic shock.
ASAIO J 2008;54:89-94. Crossref
20. Kohler K, Valchanov K, Nias G, Vuylsteke A. ECMO
cannula review. Perfusion 2013;28:114-24. Crossref
21. Tremblay LN, Slutsky AS. Ventilator-induced lung injury:
from the bench to the bedside. Intensive Care Med
2006;32:24-33. Crossref
22. Hickling KG, Walsh J, Henderson S, Jackson R. Low
mortality rate in adult respiratory distress syndrome
using low-volume, pressure-limited ventilation with
permissive hypercapnia: a prospective study. Crit Care
Med 1994;22:1568-78. Crossref
23. Amato MB, Barbas CS, Medeiros DM, et al. Effect of a
protective-ventilation strategy on mortality in the acute
respiratory distress syndrome. N Engl J Med 1998;338:347-54. Crossref
24. Ng GW, Leung AK, Sin KC, et al. Three-year experience of
using venovenous extracorporeal membrane oxygenation
for patients with severe respiratory failure. Hong Kong
Med J 2014;20:407-12.
25. Meade MO, Cook DJ, Guyatt GH, et al. Ventilation strategy
using low tidal volumes, recruitment maneuvers, and high
positive end-expiratory pressure for acute lung injury
and acute respiratory distress syndrome: a randomized
controlled trial. JAMA 2008;299:637-45. Crossref
26. Mercat A, Richard JC, Vielle B, et al. Positive end-expiratory
pressure setting in adults with acute lung injury and acute
respiratory distress syndrome: a randomized controlled
trial. JAMA 2008;299:646-55. Crossref
27. Gattinoni L, Caironi P. Refining ventilatory treatment for
acute lung injury and acute respiratory distress syndrome.
JAMA 2008;299:691-3. Crossref
28. National Heart, Lung, and Blood Institute Acute
Respiratory Distress Syndrome (ARDS) Clinical Trials
Network, Wiedemann HP, Wheeler AP, et al. Comparison
of two fluid-management strategies in acute lung injury. N
Engl J Med 2006;354:2564-75. Crossref
29. Lin CY, Chen YC, Tsai FC, et al. RIFLE classification is
predictive of short-term prognosis in critically ill patients
with acute renal failure supported by extracorporeal
membrane oxygenation. Nephrol Dial Transplant
2006;21:2867-73. Crossref
30. Smith AH, Hardison DC, Worden CR, Fleming GM, Taylor
MB. Acute renal failure during extracorporeal support in
the pediatric cardiac patient. ASAIO J 2009;55:412-6. Crossref
31. Yan X, Jia S, Meng X, et al. Acute kidney injury in adult
postcardiotomy patients with extracorporeal membrane
oxygenation: evaluation of the RIFLE classification and the
Acute Kidney Injury Network criteria. Eur J Cardiothorac
Surg 2010;37:334-8.
32. Chen H, Yu RG, Yin NN, Zhou JX. Combination of
extracorporeal membrane oxygenation and continuous
renal replacement therapy in critically ill patients: a
systematic review. Crit Care 2014;18:675. Crossref
33. Santiago MJ, Sánchez A, López-Herce J, et al. The use
of continuous renal replacement therapy in series with
extracorporeal membrane oxygenation. Kidney Int
2009;76:1289-92. Crossref
34. Symons JM, McMahon MW, Karamlou T, Parrish AR,
McMullan DM. Continuous renal replacement therapy
with an automated monitor is superior to a free-flow
system during extracorporeal life support. Pediatr Crit
Care Med 2013;14:404-8. Crossref
35. Harthan AA, Buckley KW, Heger ML, Fortuna RS, Mays K.
Medication adsorption into contemporary extracorporeal
membrane oxygenator circuits. J Pediatr Pharmacol Ther
2014;19:288-95.
36. Lemaitre F, Hasni N, Leprince P, et al. Propofol, midazolam,
vancomycin and cyclosporine therapeutic drug monitoring
in extracorporeal membrane oxygenation circuits primed
with whole human blood. Crit Care 2015;19:40. Crossref
37. Nader-Djalal N, Khadra WZ, Spaulding W, Panos AL. Does
propofol alter the gas exchange in membrane oxygenators?
Ann Thorac Surg 1998;66:298-9. Crossref
38. Oliver WC. Anticoagulation and coagulation management
for ECMO. Semin Cardiothorac Vasc Anesth 2009;13:154-75. Crossref
39. ELSO anticoagulation guidelines 2014. Ann Arbor, MI: Extracorporeal Life Support Organization; 2014.
40. Pollak U, Yacobobich J, Tamary H, Dagan O, Manor-Shulman O. Heparin-induced thrombocytopenia and
extracorporeal membrane oxygenation: a case report and
review of the literature. J Extra Corpor Technol 2011;43:5-12.
41. Sanfilippo F, Asmussen S, Maybauer DM, et al. Bivalirudin
for alternative anticoagulation in extracorporeal membrane
oxygenation: a systematic review. J Intensive Care Med
2016 Jun 29. Epub ahead of print. Crossref
42. Ranucci M, Ballotta A, Kandil H, et al. Bivalirudin-based
versus conventional heparin anticoagulation for
postcardiotomy extracorporeal membrane oxygenation.
Crit Care 2011;15:R275. Crossref
43. ELSO general guidelines for all ECLS cases. Version
1.3. Ann Arbor, MI: Extracorporeal Life Support Organization; 2013.