Hong Kong Med J 2015 Aug;21(4):299–303 | Epub 5 Jun 2015
DOI: 10.12809/hkmj144436
© Hong Kong Academy of Medicine. CC BY-NC-ND 4.0
ORIGINAL ARTICLE
Experience of more than 100 preimplantation genetic diagnosis cycles for monogenetic diseases
using whole genome amplification and linkage analysis in a single centre
Judy FC Chow, MPhil1;
William SB Yeung, PhD1;
Vivian CY Lee, FHKAM (Obstetrics and Gynaecology)2;
Estella YL Lau, PhD2;
PC Ho, FRCOG, FHKAM (Obstetrics and Gynaecology)1;
Ernest HY Ng, FRCOG, FHKAM (Obstetrics and Gynaecology)1
1 Department of Obstetrics and Gynaecology, The University of Hong
Kong, Queen Mary Hospital, Pokfulam, Hong Kong
2 Department of Obstetrics and Gynaecology, Queen Mary Hospital, Hong
Kong
Corresponding author: Dr William SB Yeung (wsbyeung@hku.hk)

Abstract
Objective: To report the outcomes of more than
100 cycles of preimplantation genetic diagnosis for
monogenetic diseases.
Design: Case series.
Setting: Tertiary assisted reproductive centre in
Hong Kong, where patients needed to pay for the
cost of preimplantation genetic diagnosis on top
of standard in-vitro fertilisation charges.
Patients: Patients undergoing preimplantation
genetic diagnosis for monogenetic diseases at the
Centre of Assisted Reproduction and Embryology,
Queen Mary Hospital–The University of Hong Kong
between 1 August 2007 and 30 April 2014 were
included.
Interventions: In-vitro fertilisation, intracytoplasmic
sperm injection, embryo biopsy, and
preimplantation genetic diagnosis.
Main outcome measures: Ongoing pregnancy rate
and implantation rate.
Results: Overall, 124 cycles of preimplantation
genetic diagnosis were initiated in 76 patients,
101 cycles proceeded to preimplantation genetic
diagnosis, and 92 cycles had embryo transfer. The
ongoing pregnancy rate was 28.2% per initiated cycle
and 38.0% per embryo transfer, giving an implantation rate of
35.2%. There were 16 frozen-thawed embryo transfer
cycles in which, following preimplantation genetic
diagnosis, cryopreserved embryos were replaced
resulting in an ongoing pregnancy rate of 37.5% and
implantation rate of 30.0%. The cumulative ongoing
pregnancy rate was 33.1%. The most frequent
indication for preimplantation genetic diagnosis
was thalassaemia, followed by neurodegenerative
disorder and cancer predisposition. There was no
misdiagnosis.
Conclusions: Preimplantation genetic diagnosis
is a reliable method to prevent couples conceiving
fetuses severely affected by known genetic disorders,
with ongoing pregnancy and implantation rates
similar to those for in-vitro fertilisation for routine
infertility treatment.
New knowledge added by this
study
- Preimplantation genetic diagnosis is feasible and reliable for at least 20 genetic conditions in Hong Kong.
- Preimplantation genetic diagnosis should be considered an alternative method in preconception counselling for couples at risk for a serious genetic disease.
Introduction
Preimplantation genetic diagnosis (PGD) refers to
the determination of genotype of embryos before
transfer during in-vitro fertilisation (IVF) cycles. The
technique can prevent couples at risk for a serious
genetic disease from having an affected fetus, and
therefore protects couples from the psychological
trauma associated with carrying an affected child,
termination of pregnancy, or recurrent miscarriage.
During PGD, one blastomere is biopsied from each
day-3 embryo (6-8 cells) and, after whole genome
amplification (WGA), mutations can be detected
directly by minisequencing and/or indirectly by
linkage analysis. The first PGD baby was born in
1989 following PGD for a sex-linked genetic disease
using polymerase chain reaction (PCR) for sex
determination.1
According to a recent report of the European
Society of Human Reproduction and Embryology
(ESHRE) PGD Consortium,2 there were more
than 4500 PGD cycles performed for monogenetic
diseases worldwide in 2002 to 2012. The Centre of
Assisted Reproduction and Embryology, Queen
Mary Hospital–The University of Hong Kong (HKU-QMH
CARE) achieved the first live birth after PGD
for alpha-thalassaemia in 2005.3 Due to the very
limited amount of DNA available for diagnosis in a
single cell, the major technical challenge of PGD is
contamination and allele dropout, which may result
in misdiagnosis. In August 2007, we adopted the
platform of WGA for PGD. Such WGA amplifies
a major portion of the genome of single cells with
good reproducibility,4 and enables direct mutation
detection and linkage analysis simultaneously for
accurate determination of the genotype of embryos.
We reported our first live birth after PGD for
Huntington’s disease (HD) using WGA in 2009.5 We
now report the outcome of over 100 PGD cycles for
monogenetic diseases at the HKU-QMH CARE.
Methods
Study population
Data from all treatment cycles were stored in a
database and PGD cases were coded for indication.
The data of all PGD cycles for monogenetic diseases
performed in the Department of Obstetrics and
Gynaecology, Queen Mary Hospital/The University
of Hong Kong from 1 August 2007 to 30 April 2014
were retrieved. Depending on the monogenetic
disease, the definitive mutation(s) responsible
for the disease were confirmed in accredited
genetic laboratories, including the Department of
Pathology, Queen Mary Hospital; Clinical Genetic
Service, Department of Health, Hong Kong SAR;
and Molecular Pathology Division, Hong Kong
Sanatorium & Hospital. Preimplantation genetic
diagnosis was offered to couples with a defined
genetic disease, irrespective of whether the couples
had a previous affected pregnancy. All couples
were extensively counselled by the reproductive
medicine subspecialists and a clinical geneticist on
the potential risks of IVF, intracytoplasmic sperm
injection (ICSI), and PGD. The couples decided
whether to proceed to PGD after non-directive
informative counselling. They were also advised
to have a confirmatory prenatal diagnosis for the
ensuing pregnancy. Depending on the availability
of the test, couples could choose an invasive or
non-invasive prenatal diagnostic test to confirm
the diagnosis by an accredited genetic laboratory
different from the PGD laboratory.
Treatment regimen
The details of the protocols for the ovarian
stimulation regimen, gamete handling, and frozen-thawed
embryo transfer (FET) have been previously
described.6 Surplus good-quality embryos
unaffected by monogenetic diseases were vitrified by
the CVM Vitrification System (CryoLogic, Mulgrave,
Australia). If the patient did not become pregnant in
the fresh cycle, the vitrified embryos were warmed
and replaced in a subsequent FET cycle.
Preimplantation genetic diagnosis
The HKU-QMH CARE has been performing PGD for
about 10 years. The procedures for PGD have been
previously described.5 In brief, embryo biopsy was
performed on day 3 at the 6 to 8 cells stage, with one
blastomere biopsied. Whole genome amplification
by multiple displacement amplification was
performed on a single blastomere.5 In all the PGD
cases for monogenetic diseases, apart from direct
mutation detection (except for those involving large
deletions), linkage analysis was performed by two
to 10 microsatellite markers flanking the mutation
to reduce possible errors due to allelic dropout.
Aneuploidy was not determined because of lack
of indications. When required, human leukocyte
antigen (HLA) typing was performed by PCR-based
sequence specific primer (Collaborative Transplant
Study, University of Heidelberg, Heidelberg,
Germany) in the same setting as for PGD.
Results
Between 1 August 2007 and 30 April 2014, 76 couples
initiated 124 cycles for monogenetic diseases. The
median age of the women was 35 (range, 26-41)
years. Embryo biopsy and PGD were performed
in 101 cycles, including three cycles of combined
HLA typing and beta-thalassaemia. The mean number of embryos biopsied per oocyte retrieval cycle was 6.1 (761/124). A total of
761 blastomeres were biopsied and a conclusive
diagnosis was obtained for 692 (91%) blastomeres.
An inconclusive diagnosis during PGD could be due
to failure in WGA or aneuploidy. Preimplantation
genetic diagnosis was cancelled in 23 (18.5%)
cycles after initiation of stimulation because
of poor responses (13 cycles), risk for ovarian
hyperstimulation syndrome (OHSS; 4 cycles), no
mature oocytes available (3 cycles), failed fertilisation
(2 cycles), or an embryologist was unavailable for
PGD (1 cycle). In case of poor response (<4 good-quality
embryos on day 3), cleavage-stage embryos
were frozen, subsequently thawed, and pooled with
fresh embryos from the following stimulated cycle
for PGD. When there was excessive ovarian response
and a patient was at risk for OHSS, all cleavage-stage
embryos were cryopreserved and PGD was
performed in the hormone replacement treatment
cycles.
Overall, 92 PGD cycles proceeded to embryo
transfer with one or two blastocysts replaced
(mean, 1.8), resulting in an ongoing pregnancy
rate (pregnancy beyond 8-10 weeks of gestation)
of 28.2% per initiated cycle, 38.0% per transfer, and
implantation rate of 35.2% (Table 1). Embryo transfer was cancelled in nine (8.9%) cycles after PGD due
to no genetically transferrable embryos available
(4 cycles), no HLA-matched embryo (1 cycle), or
patient at risk for OHSS (4 cycles). From August
2012 onwards, all genetically transferrable embryos
were cryopreserved after PGD when patients were at
risk for OHSS.
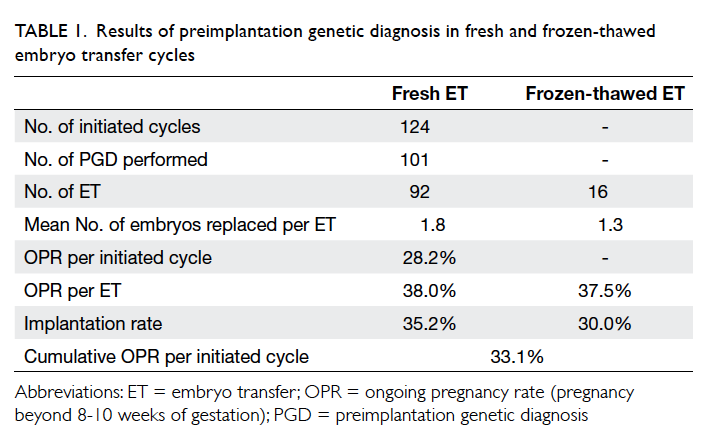
Table 1. Results of preimplantation genetic diagnosis in fresh and frozen-thawed embryo transfer cycles
There were 16 cycles of natural-cycle FET for
PGD blastocysts, resulting in an ongoing pregnancy
rate of 37.5% per transfer. The mean number of
blastocysts replaced in the FET cycle was 1.3. The
implantation rate in the FET cycle was 30.0%. The
cumulative ongoing pregnancy rate was 33.1% per
initiated cycle (Table 1).
The indications for PGD are listed in Table 2. The most frequent indication for PGD was
thalassaemia (70.2%), followed by spinocerebellar
ataxia type 3 (SCA3; 4.8%), and HD (4.0%).
Preimplantation genetic diagnosis was performed
for 20 monogenetic diseases. Successful pregnancy
was achieved after PGD for 14 genetic diseases. The
pregnant women were referred to the maternal-fetal
medicine team at Tsan Yuk Hospital for counselling
and confirmation of the genetic diseases by prenatal
diagnosis or postnatal cord blood genetic tests.
The latter was chosen by some of the patients who
worried about the risk of miscarriage associated with
invasive prenatal tests. Based on the available results
of the confirmation genetic tests, no misdiagnosis
was found in this small series.
Discussion
In 2014, the ESHRE PGD Consortium published
data for 1597 PGD cycles from 60 PGD centres in
Europe in 2009.7 When comparing our PGD data
with those of the ESHRE PGD Consortium, we have
a comparable mean number of embryos biopsied per
oocyte retrieval cycle (6.1 vs 6.6) and similar mean
number of embryo transfers per retrieval cycle (1.4
vs 1.3). The ESHRE PGD Consortium reported a
clinical pregnancy rate of 30.2% per transfer, while
the ongoing pregnancy rate per transfer in our centre
was 38.0% for fresh transfer and 37.5% for FET. The
ongoing pregnancy rate per transfer in the IVF-ICSI
cycle without PGD was 36% in our centre. The
implantation rate of PGD embryos from the ESHRE
PGD Consortium was 21.3% and in our programme
was 35.2% for fresh cycles and 30.0% for FET cycles.
The implantation rate in our IVF-ICSI programme
without PGD was 27.7%.
A limitation of the present study was that the
number of cases of each genetic disease involved
was small. Many genetic diseases had only one case
in this cohort of patients. The usefulness of PGD in
these cases needs to be confirmed in a larger cohort
of patients.
The cancellation rate for PGD after initiation
of stimulation was 18.5% (23/124). The major reason
for cancellation was poor response leading to a small
number of good-quality embryos available for PGD.
In such circumstance, cleavage-stage embryos were
frozen and batched for the next stimulated PGD
cycle. By increasing the number of embryos tested
per PGD cycle, this ‘batching’ strategy increased the
chance of having disease-free embryos for transfer.
The strategy also enabled patients to have the best-quality
embryo chosen, instead of experiencing
multiple cancellations of embryo transfer after PGD.
For those cases proceeding to PGD, 8.9%
resulted in no embryo transfer. Embryo transfer
was cancelled because of the risk for OHSS or no
genetically transferable embryos available. In cases
at risk for OHSS, good-quality blastocysts were
vitrified for subsequent FET cycles. Some reports
have suggested that FET cycles may result in higher
pregnancy and implantation rates than stimulated
cycles8 9 10 due to the better receptivity of the
endometrium without gonadotropin stimulation. An
additional benefit of FET lies in being free of risk for
OHSS when compared with fresh embryo transfer.
Among the 124 cycles initiated for PGD during
the study period, alpha- and beta-thalassaemia
(autosomal recessive disorders) accounted for
70.2% of the PGD cases. This is due to a high
percentage of carriers in the population in Hong
Kong, with a prevalence of 4.5% and 2.8% for alpha-thalassaemia
(South-East Asian deletion type) and
beta-thalassaemia, respectively.11 12 The second most
frequent indication for PGD was neurodegenerative
disorders such as SCA3 and HD, which are inherited
in an autosomal dominant fashion. These conditions
accounted for 8.9% of PGD cycles. The local
prevalence of HD is estimated to be four per million
population.13 14 There were 16 SCA cases diagnosed
in three hospitals in Hong Kong within 3 years, and
SCA3 accounted for 75% of the cases.15
It is noteworthy that 4.8% of the PGD cases
were for cancer predisposition. However, it is always
controversial to offer PGD to couples with inherited
mutations of late-onset reduced penetrance cancer
predisposition such as breast cancer.16 Additional
controversy lies in the fact that PGD does not
remove all the risks associated with the disease.
Other known risk factors involved in breast cancer
include obesity, use of hormone therapies (progestin
and oestrogen), increased breast tissue density,
alcohol use, and physical inactivity.17 In 2003, the
ESHRE Ethics Task Force published a recommended
multidisciplinary approach to the application of PGD,
stating that PGD for multifactorial diseases such as
BRCA mutation is acceptable notwithstanding the
uncertainties about the genetic predisposition and
the epigenetic influence.18 The United Kingdom
Human Fertilisation and Embryology Authority
also accepted PGD for various hereditary cancer
syndromes, including familial adenomatous
polyposis, neurofibromatosis type 1 and type 2, and
von Hippel–Lindau syndrome.
Apart from the monogenetic diseases, other
genetic tests such as HLA typing can be done on the
same samples. In these cases, the embryos were not
treated differently from those without HLA typing.
Therefore, it is unlikely that the additional test would
affect the ongoing pregnancy rate or implantation
rate. Without indication, aneuploidy screening
was not performed at the same time as PGD for
monogenetic diseases. In our series, there were two
pregnancies complicated by aneuploidy (trisomy
21 and trisomy 13 for each). Both patients were young
(age, 31 years) with no previous pregnancy affected
by aneuploidy. The usefulness of preimplantation
aneuploidy screening in young patients is still under
debate.
In our centre, we always consider each case
individually and take into account the family
histories of the couples, especially for late-onset
genetic diseases such as SCA3 and HD and those
with reduced penetrance and multifactorial cancer
predisposition such as breast cancer. Patients were
extensively counselled by a geneticist before referral
for PGD treatment. Informative and non-directive
counselling by specialists in reproductive medicine
was also given to patients. Options other than
PGD—such as prenatal diagnosis after becoming
pregnant without PGD, gamete donation, embryo
donation, adoption, or remaining childless—were
discussed. Patients who became pregnant after PGD
were referred to the prenatal diagnosis centre in Tsan
Yuk Hospital for counselling. Prenatal diagnosis was
encouraged for confirmation of the genetic status
of the fetus. If patients refused to undergo invasive
prenatal testing, postnatal cord blood genetic
confirmation may be considered if the offspring
will benefit from the early surveillance or postnatal
treatment. When encountering controversial PGD
cases, meetings were held to discuss the case with
geneticists, obstetricians, specialists in prenatal
diagnosis, ethicists, and other relevant specialists
such as oncologists before offering PGD.
Conclusions
Preimplantation genetic diagnosis is a reliable
method with ongoing pregnancy rate and
implantation rate similar to those with IVF and
ICSI. When couples have known genetic diseases,
they should be counselled before pregnancy for
preimplantation genetic diseases as an alternative
to prenatal diagnosis, even when they do not have a
previous affected pregnancy.
Acknowledgements
We would like to thank the patients, nurses,
clinicians, technicians, and embryologists at the
Centre of Assisted Reproduction and Embryology,
Queen Mary Hospital–The University of Hong Kong
for their contribution in the PGD programme.
Declaration
The authors declare that they have no conflict of interest.
References
1. Handyside AH, Kontogianni EH, Hardy K, Winston
RM. Pregnancies from biopsied human preimplantation
embryos sexed by Y-specific DNA amplification. Nature
1990;344:768-70. Crossref
2. Harper JC, Wilton L, Traeger-Synodinos J, et al. The
ESHRE PGD Consortium: 10 years of data collection. Hum
Reprod Update 2012;18:234-47. Crossref
3. Chan V, Ng EH, Yam I, Yeung WS, Ho PC, Chan TK.
Experience in preimplantation genetic diagnosis for
exclusion of homozygous alpha degrees thalassemia.
Prenat Diagn 2006;26:1029-36. Crossref
4. Hellani A, Coskun S, Benkhalifa M, et al. Multiple
displacement amplification on single cell and possible PGD
applications. Mol Hum Reprod 2004;10:847-52. Crossref
5. Chow JF, Yeung WS, Lau EY, et al. Singleton birth after
preimplantation genetic diagnosis for Huntington disease
using whole genome amplification. Fertil Steril 2009;92:828.e7-10. Crossref
6. Ng EH, Yeung WS, Lau EY, So WW, Ho PC. High
serum oestradiol concentrations in fresh IVF cycles do not
impair implantation and pregnancy rates in subsequent
frozen-thawed embryo transfer cycles. Hum Reprod
2000;15:250-5. Crossref
7. Moutou C, Goossens V, Coonen E, et al. ESHRE PGD
Consortium data collection XII: cycles from January to
December 2009 with pregnancy follow-up to October
2010. Hum Reprod 2014;29:880-903. Crossref
8. Evans J, Hannan NJ, Edgell TA, et al. Fresh versus frozen
embryo transfer: backing clinical decisions with scientific
and clinical evidence. Hum Reprod Update 2014;20:808-21. Crossref
9. Roque M, Lattes K, Serra S, et al. Fresh embryo transfer
versus frozen embryo transfer in in vitro fertilization
cycles: a systematic review and meta-analysis. Fertil Steril
2013;99:156-62. Crossref
10. Shapiro BS, Daneshmand ST, Garner FC, Aguirre M,
Hudson C. Clinical rationale for cryopreservation of
entire embryo cohorts in lieu of fresh transfer. Fertil Steril
2014;102:3-9. Crossref
11. Lau YL, Chan LC, Chan YY, et al. Prevalence and genotypes
of alpha- and beta-thalassemia carriers in Hong Kong—implications for population screening. N Engl J Med
1997;336:1298-301. Crossref
12. Sin SY, Ghosh A, Tang LC, Chan V. Ten years’ experience
of antenatal mean corpuscular volume screening and
prenatal diagnosis for thalassaemias in Hong Kong. J
Obstet Gynaecol Res 2000;26:203-8. Crossref
13. Leung CM, Chan YW, Chang CM, Yu YL, Chen CN.
Huntington’s disease in Chinese: a hypothesis of its origin.
J Neurol Neurosurg Psychiatry 1992;55:681-4. Crossref
14. Chang CM, Yu YL, Fong KY, et al. Huntington’s disease in
Hong Kong Chinese: epidemiology and clinical picture.
Clin Exp Neurol 1994;31:43-51.
15. Lau KK, Lam K, Shiu KL, et al. Clinical features of
hereditary spinocerebellar ataxia diagnosed by molecular
genetic analysis. Hong Kong Med J 2004;10:255-9.
16. Konstantopoulou I, Pertesi M, Fostira F, Grivas A,
Yannoukakos D. Hereditary cancer predisposition
syndromes and preimplantation genetic diagnosis: where
are we now? J BUON 2009;14 Suppl 1:S187-92.
17. Emens LA, Jaffee EM. Leveraging the activity of tumor
vaccines with cytotoxic chemotherapy. Cancer Res
2005;65:8059-64. Crossref
18. Shenfield F, Pennings G, Devroey P, Sureau C, Tarlatzis
B, Cohen J; ESHRE Ethics Task Force. Taskforce 5:
preimplantation genetic diagnosis. Hum Reprod
2003;18:649-51. Crossref