Hong Kong Med J 2014 Dec;20(6):474–80 | Epub 24 Oct 2014
DOI: 10.12809/hkmj144242
© Hong Kong Academy of Medicine. CC BY-NC-ND 4.0
ORIGINAL ARTICLE CME
Subthalamic nucleus deep brain stimulation for Parkinson’s disease: evidence for effectiveness and limitations from 12 years’ experience
Movement Disorder Group, Prince of Wales Hospital, The Chinese
University of Hong Kong, Shatin, Hong Kong;
Anne YY Chan, MB, ChB, MRCP1;
Jonas HM Yeung, MB, ChB, MRCP2;
Vincent CT Mok, MD1;
Vincent HL Ip, MB, ChB, MRCP1;
Adrian Wong, PhD1;
SH Kuo, MD3;
Danny TM Chan, FRCS (Edin), FHKAM (Surgery)4;
XL Zhu, FRCS (Edin), FHKAM (Surgery)4;
Edith Wong, BSc4;
Claire KY Lau, MSc4;
Rosanna KM Wong, MSc5;
Venus Tang, PhD4,6;
Christine Lau, MSc1;
WS Poon, FHKAM (Medicine)4
1 Division of Neurology, Department of Medicine and Therapeutics, Prince
of Wales Hospital, The Chinese University of Hong Kong, Hong Kong
2 Division of Neurology, Department of Medicine, Alice Ho Miu Ling
Nethersole Hospital, Hong Kong
3 Neurological Institutes of New York, Columbia University, United States
4 Division of Neurosurgery, Department of Surgery, Prince of Wales
Hospital, The Chinese University of Hong Kong, Hong Kong
5 Department of Occupational Therapy, Prince of Wales Hospital, The
Chinese University of Hong Kong, Hong Kong
6 Department of Clinical Psychology, Prince of Wales Hospital, Hospital
Authority, Hong Kong
Corresponding author: Prof WS Poon (wpoon@cuhk.edu.hk)

Abstract
Objective: To present the result and experience
of subthalamic nucleus deep brain stimulation for
Parkinson’s disease.
Design: Case series.
Setting: Prince of Wales Hospital, Hong Kong.
Patients: A cohort of patients with Parkinson’s
disease received subthalamic nucleus deep brain
stimulation from September 1998 to January
2010. Patient assessment data before and after the
operation were collected prospectively.
Results: Forty-one patients (21 male and 20 female)
with Parkinson’s disease underwent bilateral
subthalamic nucleus deep brain stimulation and
were followed up for a median interval of 12 months.
For the whole group, the mean improvements of
Unified Parkinson’s Disease Rating Scale (UPDRS)
parts II and III were 32.5% and 31.5%, respectively
(P<0.001). Throughout the years, a multidisciplinary
team was gradually built. The deep brain stimulation
protocol evolved and was substantiated by updated
patient selection criteria and outcome assessment,
integrated imaging and neurophysiological targeting,
refinement of surgical technique as well as the
accumulation of experience in deep brain stimulation
programming. Most of the structural improvement
occurred before mid-2005. Patients receiving the
operation before June 2005 (19 cases) and after
(22 cases) were compared; the improvements in
UPDRS part III were 13.2% and 55.2%, respectively
(P<0.001). There were three operative complications
(one lead migration, one cerebral haematoma, and
one infection) in the group operated on before 2005.
There was no operative mortality.
Conclusions: The functional state of Parkinson’s
disease patients with motor disabilities refractory
to best medical treatment improved significantly
after subthalamic nucleus deep brain stimulation. A
dedicated multidisciplinary team building, refined
protocol for patient selection and assessment,
improvement of targeting methods, meticulous
surgical technique, and experience in programming
are the key factors contributing to the improved
outcome.
New knowledge added by this
study
- The current study provides data on subthalamic nucleus deep brain stimulation (DBS) for Parkinson’s disease from a tertiary hospital in Hong Kong and the development of this treatment method over the past 12 years.
- There was significant improvement in the outcome between the early and later groups of the series. Possible factors contributing to the improvement are dedicated multidisciplinary team building, refinement of the DBS protocol for patient assessment and selection, improvement of targeting methods, meticulous surgical technique, and accumulation of experience in DBS programming.
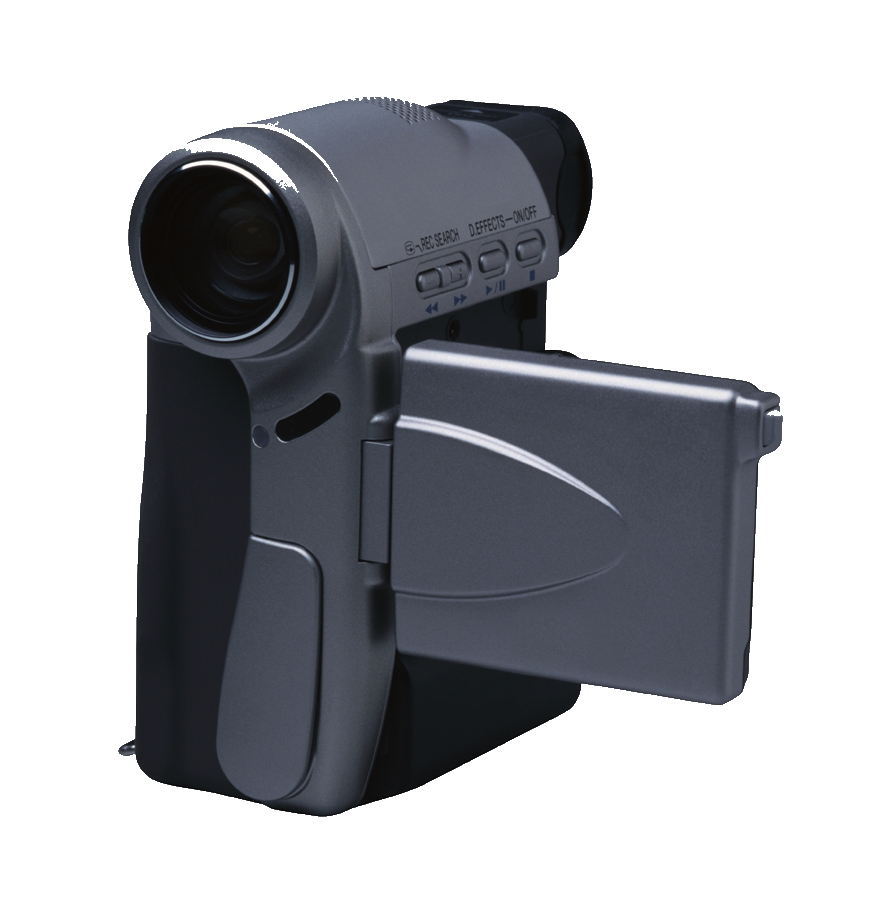
Introduction
Parkinson’s disease (PD) is the second most common
neurodegenerative disorder that initially affects the
dopaminergic system and eventually involves other
neurotransmitter systems, with an unrelenting
course. It is well known that motor fluctuations like
wearing-off and peak-dose dyskinesia are common
motor complications few years after patients
are started on medical treatment. When these
complications become very disabling despite maximal
adjustment of pharmacological agents, deep brain
stimulation (DBS) has been shown to be effective and
safe with lasting benefits for at least up to 10 years.1 2 3 4 5
Deep brain stimulation is a neurosurgical intervention
that entails inserting microelectrodes with imaging
and neurophysiological guidance at targeted regions,
such as the subthalamic nucleus (STN) or globus
pallidus interna (GPi) in order to achieve alleviation
of most of the motor complications. We studied
DBS performed in a cohort of PD patients in a single
Hong Kong centre over a period of 12 years. Patients’
outcomes improved significantly throughout all these
years. Here, we review our results of STN DBS for
PD and summarise our experience, with particular
emphasis on evaluating the effectiveness and safety
of this novel treatment.
Methods
Patients
Patients who fulfilled the Queen Square Brain
Bank Criteria for diagnosis of PD and experienced
significant disability due to motor fluctuations
despite maximal medical therapy were admitted to
the Prince of Wales Hospital and jointly evaluated
for the suitability for DBS by a Movement Disorder
Group composed of neurologists, neurosurgeons,
and a nurse specialist. After June 2005, this
multidisciplinary group was further developed
to include a radiologist, clinical psychologist,
occupational therapist, physiotherapist, and speech
therapist. The assessment comprised a predefined
protocol, which was continuously updated over the
years. This assessment protocol included clinical
assessment, levodopa challenge test, video recording,
neuroimaging, and neurocognitive and psychiatric
evaluations. Patients who received STN DBS from
September 1998 (the first patient who received STN
DBS in our centre) to January 2010 were included
in the present study. Assessment data were obtained
before and after the operation (medication-off
state and stimulator-on) and included the Unified
Parkinson’s Disease Rating Scale (UPDRS), modified
Hoehn-and-Yahr stage (both at PD off-state),
levodopa equivalent dose, body weight, and patients’
diaries at preoperation and at least 2 months
postoperation.
Surgical information
All patients had stereotactic guidance for the
insertion of electrodes under local anaesthesia.
Microelectrode recording (MER) technique was
introduced since year 2000. Frame-based stereotaxy
was performed in most of the patients except three
who underwent frameless stereotaxy (2005) with
equally accurate targeting. Before 2003, an old
version of Zeppelin magnetic resonance imaging
(MRI)–compatible frame was used, which was not
compatible with the computer planning system
available at that time. Since 2003, a Leksell G frame (Elekta AB, Stockholm, Sweden)
was acquired together with a computer planning
system (iPlan; Brain LAB, Feldkirchen, Germany) for
image targeting and trajectory planning. With these,
the image targeting and trajectory were planned
based on preoperative MRI. On the day of operation,
a computed tomography (CT) scan of brain with the
stereotactic frame fixed to the head was performed
and fused to the MRI plan in the computer planning
system. Such a practice gave us more time for image
targeting and shortened the time used for MRI
and target planning on the day of operation before
entering the operating theatre; this contributed to
the accuracy of STN targeting and patient comfort. A
dedicated MRI protocol was established since 2008,
which ensured the consistency of high-quality MRI
for targeting. In 2003, microdriver was introduced for
MER which provided sub-millimetre advancement
of the microelectrode to obtain more details of
neuronal discharges and, thus, better quality of MER
for neurophysiological targeting. Macrostimulation
was performed in all patients for target confirmation.
Deep brain stimulation electrode was implanted if
satisfactory signals from MER and response from
macrostimulation were obtained. For anchoring
the DBS lead at the burr-hole site, a reliable device
(Navigus; Medtronic, Minneapolis [MN], US) was
introduced in 2002. The Pulse Generator (Itrel
II or Soletra for unilateral, Kinetra for bilateral;
Medtronic, Minneapolis [MN], US) was implanted
under general anaesthesia. The DBS was usually
switched on within 2 weeks after the operation. A
nurse specialist took up DBS programming under
the supervision of a neurologist and neurosurgeon
in 2004, which facilitated experience accumulation
and consistency in DBS programming.
Statistical analyses
Descriptive data were expressed as mean ± standard
deviation (SD). Wilcoxon test was used to compare
scores of modified Hoehn-and-Yahr stage, levodopa
equivalent dose, body weight, patients’ diaries, as
well as scores of individual items of activities of daily
living (part II) and motor examination (part III) of
UPDRS before and after operation. Tests with a P
value of ≤0.05 were considered to be statistically
significant. Statistical analyses were performed
with the Statistical Package for the Social Sciences
(Windows version 15.0; SPSS Inc, Chicago [IL], US).
Results
Between September 1998 and January 2010, a total of
51 PD patients received DBS. Of them, six received
unilateral STN DBS, two received GPi DBS, and
two received nucleus ventralis intermedius (VIM)
stimulation. Overall, 41 patients received bilateral
STN DBS (21 male, 20 female; age at operation:
mean 54, SD 7, range 40-71
years). All were Han Chinese except one who was of
Portuguese ethnicity. Mean (± SD) duration of PD at
operation was 10 ± 4 (range, 3-22) years (Table 1).
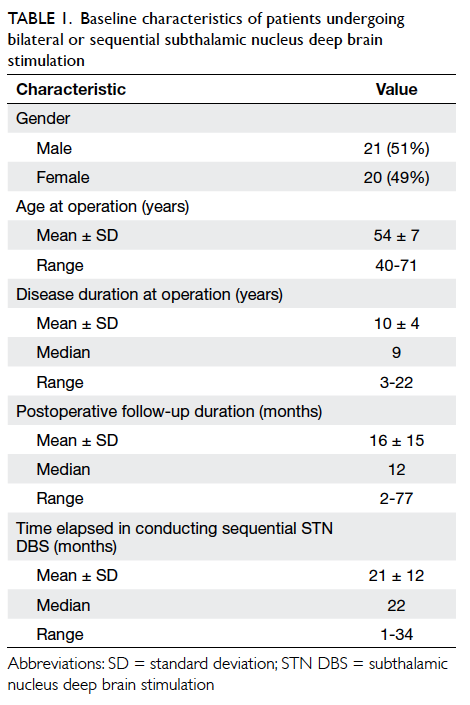
Table 1. Baseline characteristics of patients undergoing bilateral or sequential subthalamic nucleus deep brain stimulation
There were three complications, namely, lead
migration, infection, and cerebral haematoma;
all occurred in 2001. Lead migration occurred in
2001 three months after the operation. The patient
complained of increased PD symptoms after a fall on
level ground. One DBS lead was found withdrawn
from the target. It was related to the insecurity of
lead fixation. Revision operation was done for the
case with good recovery. Infection occurred in a case
3 weeks after DBS operation in 2001. Apart from
abscess formation in the chest wall where the pulse
generator was implanted, MRI brain showed contrast
enhancement around the DBS electrodes. All the
hardware including the pulse generator and the
DBS leads were removed and the patient received a
course of antibiotics. He received DBS again in 2006
and enjoyed good effect. Cerebral haematoma also
occurred in 2001. The patient became drowsy during
the operation. The surgery was stopped and CT
brain revealed a subcortical haematoma likely related
to venous infarction. Craniotomy for haematoma
evacuation was performed. The patient recovered but
with residual hemiparesis. There was no mortality.
At postoperative follow-up with a median
assessment time of 12 months, both UPDRS parts
II and III showed improvements of 32.5% and 31.5%,
respectively (P<0.001) in the whole study sample (Table 2). In view of the evolvement of the protocol, improvement in targeting methods, refinement of surgical
technique as well as accumulation of programming experience over the 12 years, the improvement
rates in patients who had DBS from 1999 to May 2005 and from June 2005 to 2010 were analysed
separately and compared. Patients in the later group had significantly higher rate of improvement than
those in the early group (Table 3). The improvement in UPDRS part II and part III for the two groups were 5.1% vs 55.0% (P=0.005) and 13.2% vs 55.2%,
respectively (P<0.001). The Figure shows the percentage of improvement in UPDRS parts II and III. There was statistically significant increase in body
weight after operation as shown in Table 2. However, there was a non-significant trend with regard to the reduction of levodopa-equivalent daily dose after surgery. A comprehensive neurocognitive evaluation was established after 2008 (Table 4). Three patients completed pre- and post-operative evaluation. Their functioning level in various domains remained unaffected at postoperative evaluation, although a reduction in verbal fluency was noted. No separate statistical analysis was performed due to the limited
number of subjects.
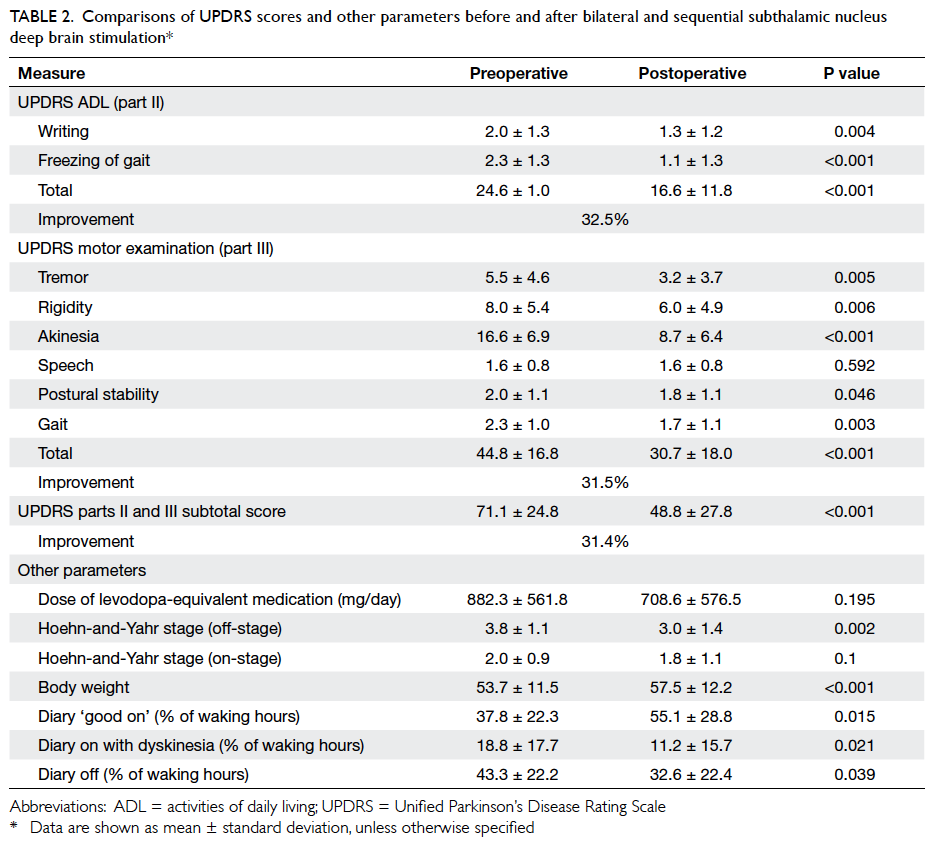
Table 2. Comparisons of UPDRS scores and other parameters before and after bilateral and sequential subthalamic nucleus deep brain stimulation
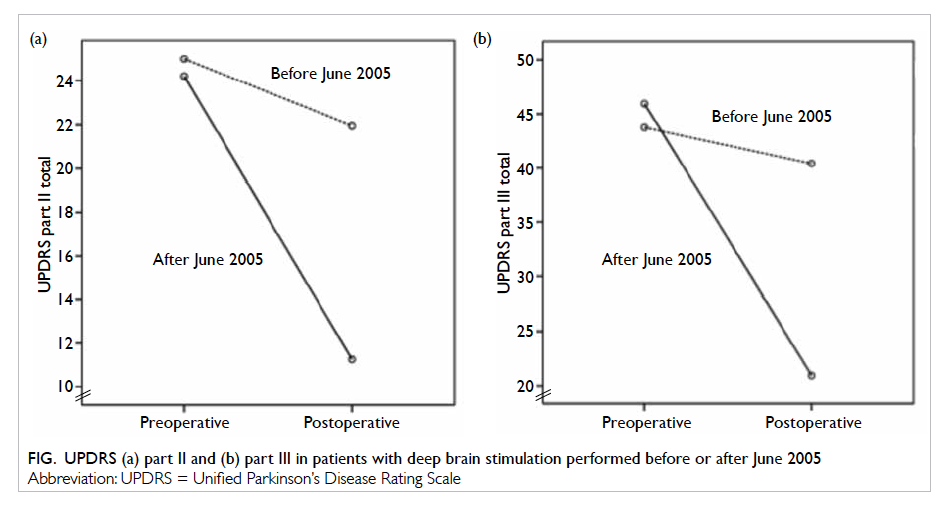
Figure. UPDRS (a) part II and (b) part III in patients with deep brain stimulation performed before or after June 2005
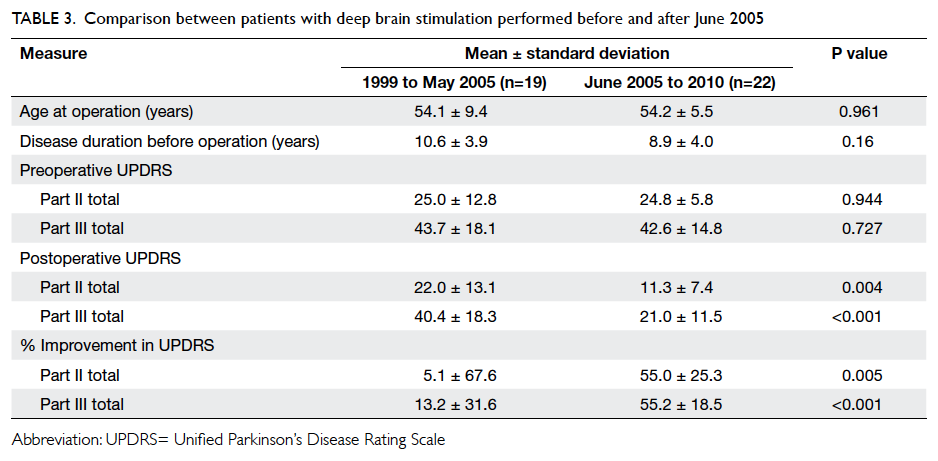
Table 3. Comparison between patients with deep brain stimulation performed before and after June 2005
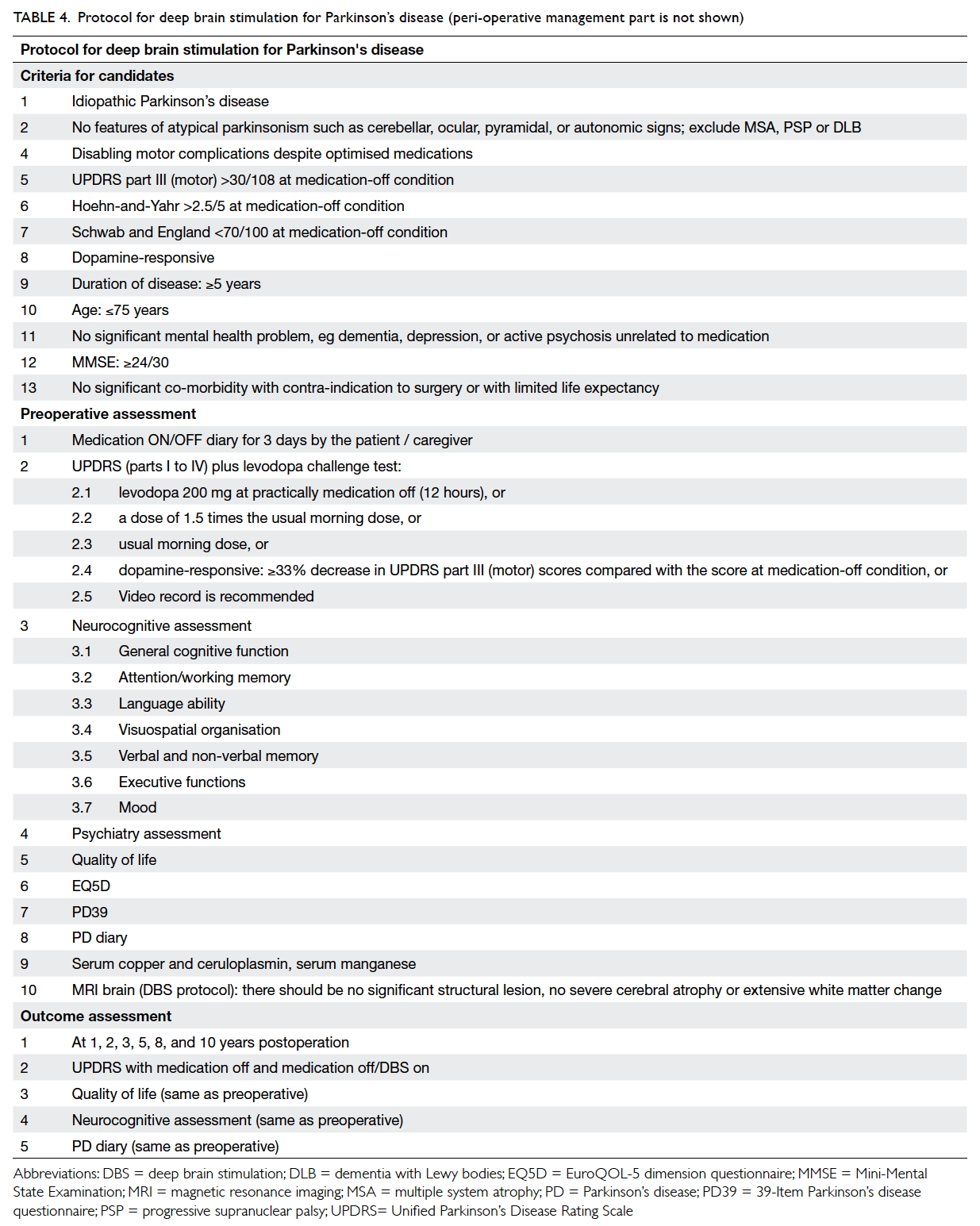
Table 4. Protocol for deep brain stimulation for Parkinson’s disease (peri-operative management part is not shown)
As per the patients’ own diary reporting, there were statistically significant improvements in “on-period without dyskinesia” (ie more ‘good-on’), “on-period with dyskinesia”, and “off-period” in terms of percentage of waking hours (Table 2).
Discussion
Parkinson’s disease is a neurodegenerative condition
typically manifesting in an unrelenting progressive
course. Patients often show dramatic response
to pharmacological treatments initially, but with
time, motor complications like motor fluctuations
with wearing-off or peak-dose dyskinesia occur,
eventually leading to progressive disability. Deep
brain stimulation has evolved as an important and
established treatment option for advanced PD. The
mechanism of DBS is generally believed to modulate
the circuit function by inhibition or excitation
through controlled electrical stimulation of different
targets along the circuit. Subthalamic nucleus is the
most often used target for PD. The benefits of DBS
compared with ablative surgery include its non-destructive
nature, reversibility, and adjustability.
The procedure is mainly indicated for PD patients who
are dopamine-responsive but with disabling motor
complications such as motor fluctuation, dyskinesia,
or intolerable side-effects of anti-PD medications.6
To date, there are controversies regarding the use
of DBS in the early stage of PD, and most centres
will offer this treatment for patients with significant
motor complications refractory to maximal drug
treatment. Convincing evidence shows that DBS
is an effective treatment for PD patients and the
benefits may last for at least 10 years.
Our study attempted to investigate the
effectiveness and safety of bilateral STN DBS in
PD patients who had disabilities refractory to best-available
medical treatment. The study extends over
a period of 10 years, trying to evaluate PD patients
who received bilateral STN DBS. The study excluded
patients receiving unilateral STN, as well as VIM
or GPi DBS, so that the patient group was more
homogeneous in the surgical therapeutic sense.
The median timing of postoperative assessment
was 12 months (range, 2-77 months), and it
demonstrated significant improvement in almost
all parameters in UPDRS part II and part III, except
for speech. Although our assessments were not
performed at the same time intervals for all patients
postoperatively, the results are still in concordance
with findings in published studies evaluating efficacy
of DBS at 6 months and 5 years postoperatively.
Speech was also reported to be the only dysfunction
that did not improve with STN DBS in published
cohorts in general. The exact reason for this finding
is yet to be unfolded; a recent paper suggested that
speech impairment may be related to unintended
activation of dorsal premotor cortex during STN
stimulation,7 but it echoes with the observation that
DBS improves symptoms which respond to levodopa;
speech is, however, not one of those.
The UPDRS part II on activities of daily living
indicated significant improvement, in terms of total
rating, writing, and freezing of gait. Apart from
objective assessment, patients’ self-evaluation from
their own PD diary also revealed improvements
in terms of more ‘good-on’ state, mobile without
disabling dyskinesia, as well as less off-period
compared with those after DBS.
Since our first case of DBS for PD in 1998, a
multidisciplinary team comprising a neurologist,
neurosurgeon, nurse specialist, radiologist, clinical
psychologist, occupational therapist, physiotherapist,
and speech therapist was gradually built up. From
2000 to 2004, important evolvement of our DBS
protocol included: a monthly Combined Movement
Disorders outpatient clinic run by a neurologist,
neurosurgeon, and nurse specialist; regular case
conference; dopamine challenge test; MER;
acquirement of a stereotactic frame together with a
computer planning system for imaging targeting and
trajectory planning; and appointment of a dedicated
person for DBS programming. Our experience also
increased considerably in the areas of systematic
auditing of targeting accuracy, surgical complications,
and clinical outcome.5 All these serve to explain the
significant improvements in the outcomes after 2005
as shown in the Figure. Our current DBS protocol
since 2009 for PD is shown in Table 4.
We found a non-significant trend in reduction
in the dose of anti-Parkinsonian medications in
terms of levodopa-equivalent medications after
STN DBS. This possible reduction in medication
usage is a common finding in previously published
reports3 and indicates increased independence from
pharmacological treatment after the surgery. We also
showed that weight gain was significant after STN
DBS, which is consistent with findings in the current
literature. There have been plenty of studies looking
into the possible mechanisms,8 but the exact causes
remain uncertain. Preliminary data show that weight
gain might even be more commonly encountered in
STN as compared with GPi DBS in PD, suggesting
additional factors in STN stimulation.9
The complication rate in our study is
comparable with that in another DBS centre with
8.6% hardware-related complication.10
There are limitations in our study. This was not
a prospective study. Although the data were collected
prospectively, the protocol itself was continuously
evolved. Our patients were not assessed uniformly
at the exact same time-points postoperatively; the
timing of postoperative evaluation ranged from
2 months to 77 months (mean ± SD, 17.0 ± 15.4
months), which is a rather wide range. This was
related to the logistic arrangements for patients to
have overnight admission for ensuring 12 hours
off-medication and availability of beds in a busy
tertiary emergency hospital. Quality of life (eg
39-item Parkinson’s disease questionnaire) is an
important component of outcome assessment but it
was not used in this cohort. There was no systematic
neuropsychology assessment until 2008. Despite
all these shortcomings, our study is the first series
in Hong Kong reporting the treatment outcomes
as well as the experience gained over 12 years in
bilateral STN DBS for PD patients.
Conclusions
Bilateral STN DBS for PD patients having motor
disabilities refractory to medical treatment showed
significant improvement in motor performance
and functional state, except for speech, during
an observation period of up to 77 months. The
surgical procedure was shown to be safe, with no
perioperative mortality. Patients were found to
consume less anti-Parkinsonian medications and
reported less dyskinesia, but had increased body
weight. A dedicated multidisciplinary team building,
refinement of protocol for patient assessment and
selection, improvement of targeting methods,
meticulous surgical technique, and experience in
programming are the key factors that contributed to
the improved outcomes.
References
1. Chan DT, Mok VC, Poon WS, Hung KN, Zhu XL. Surgical management of Parkinson’s disease: a critical review. Hong Kong Med J 2001;7:34-9.
2. Moro E, Lozano AM, Pollak P, et al. Long-term results of a multicenter study on subthalamic and pallidal stimulation in Parkinson’s disease. Mov Disord 2010;25:578-86. CrossRef
3. Schüpbach WM, Chastan N, Welter ML, et al. Stimulation of the subthalamic nucleus in Parkinson’s disease: a 5 year follow up. J Neurol Neurosurg Psychiatry 2005;76:1640-4. CrossRef
4. Krack P, Batir A, Van Blercom N, et al. Five-year follow-up of bilateral stimulation of the subthalamic nucleus in advanced Parkinson’s disease. N Engl J Med 2003;349:1925-34. CrossRef
5. Chan DT, Zhu XL, Yeung JH, et al. Complications of deep brain stimulation: a collective review. Asian J Surg 2009;32:258-63. CrossRef
6. Zhou JY, Yu Y, Zhu XL, Ng CP, Lu G, Poon WS. Parkinson’s disease: insights from the laboratory and clinical therapeutics. In: Nagata T, editor. Senescence. InTech 2012; 587-616. CrossRef
7. Narayana S, Jacks A, Robin DA, et al. A noninvasive imaging approach to understanding speech changes following deep brain stimulation in Parkinson’s disease. Am J Speech Lang Pathol 2009;18:146-61. CrossRef
8. Montaurier C, Morio B, Bannier S, et al. Mechanisms of body weight gain in patients with Parkinson’s disease after subthalamic stimulation. Brain 2007;130:1808-18. CrossRef
9. Sauleau P, Leray E, Rouaud T, et al. Comparison of weight gain and energy intake after subthalamic versus
pallidal stimulation in Parkinson’s disease. Mov Disord 2009;24:2149-55. CrossRef
10. Baizabal Carvallo JF, Mostile G, Almaguer M, Davidson A, Simpson R, Jankovic J. Deep brain stimulation hardware complications in patients with movement disorders: risk factors and clinical correlations. Stereotact Funct
Neurosurg 2012;90:300-6. CrossRef