DOI: 10.12809/hkmj165057
© Hong Kong Academy of Medicine. CC BY-NC-ND 4.0
MEDICAL PRACTICE
Clinical management of sepsis
SM Lam, MB, BS, FHKAM (Medicine)1;
Arthur CW Lau, MB, BS, FHKAM (Medicine)1; Rex PK Lam, MB, BS, FHKAM (Emergency Medicine)2; WW Yan, MB, BS, FHKAM (Medicine)1
1 Department of Intensive Care, Pamela Youde Nethersole Eastern
Hospital, Chai Wan, Hong Kong
2 Emergency Medicine Unit, Li Ka Shing Faculty of Medicine, The
University of Hong Kong, Pokfulam, Hong Kong
Corresponding author: Dr SM Lam (lamsm2@ha.org.hk)

Abstract
Sepsis is a common cause of hospital admission
worldwide and contributes significantly to morbidity
and mortality. The definition of sepsis has evolved
from the 1991 American College of Chest Physicians/Society of Critical Care Medicine definition
based on the criteria of systemic inflammatory
response syndrome, to the 2016 Sepsis-3 definition
that incorporates the Sequential Organ Failure
Assessment score. The landmark trial on protocolised
early goal-directed therapy was published in 2001,
but three subsequent multicentre randomised
controlled trials (ProCESS, ARISE, and ProMISe)
in 2014-2015 did not confirm a survival benefit with
protocolised care. Over the years, there has been
considerable improvement in sepsis outcome and
management that hinges on early detection; timely
source control; prompt, appropriate, and correctly
dosed antibiotics; aggressive fluid resuscitation; and
shock reversal. These are all directed by repeated
bedside assessment. This article summarises recent
developments and landmark trials that should guide
current sepsis management.
Introduction
Sepsis is a common cause of hospital admission
worldwide. The annual incidence of sepsis has
been reported to be approximately 300 to 1031 per
100 000 population in the US, and is increasing.1 In-hospital
mortality, however, decreased from 35% in
2000 to <20% in 2013.2 Numerous studies have been
performed or are ongoing in this field. The following
discussion provides an update on the change to
sepsis definition, three recent trials on protocolised
early goal-directed therapy (EGDT), and individual
components of sepsis management.
Defining and recognising sepsis:
from systemic inflammatory
response syndrome to Sequential
Organ Failure Assessment score
and the role of biomarkers
In 1991, sepsis was defined as fulfilling two or
more than two systemic inflammatory response
syndrome (SIRS) criteria in the presence of infection
(Table 1).3 Many seriously infected patients (eg old
or immunocompromised), however, are unable to
mount a SIRS. Using SIRS criteria to define severe
sepsis will miss one in eight otherwise similar
patients with substantial mortality.2 In addition, the
mortality risk has been shown to increase linearly
with each additional SIRS criterion and there is no
transition point noted at a threshold of two SIRS
criteria.
To acknowledge the above shortcomings, the
Sepsis-3 (Third International Consensus Definitions
for Sepsis and Septic Shock) in 2016 redefined sepsis
as “life-threatening organ dysfunction caused by
a dysregulated host response to infection”.4 Organ
dysfunction is identified as “an acute change in
the total Sequential Organ Failure Assessment
(SOFA) score of ≥2 points” (Table 1). In addition,
a quick SOFA (qSOFA) score was introduced for
bedside screening. Meeting two or more than two
qSOFA criteria (respiratory rate ≥22/min, altered
mentation with a Glasgow Coma Scale score of <15,
systolic blood pressure ≤100 mm Hg) should prompt
consideration of possible infection in undiagnosed
patients, investigation for organ dysfunction that
defines sepsis in infected patients, and initiation
of sepsis management where appropriate.4 Despite
these recent changes in definition, clinicians should
maintain a high index of suspicion and always
consider sepsis as a possible explanation/diagnosis
when faced with new-onset organ dysfunction in a
patient.
Apart from clinical assessment, various serum
biomarkers have been studied for their potential
role in early diagnosis of sepsis. C-reactive protein is
commonly used but it has a low specificity for sepsis.
Procalcitonin (PCT) is a prohormone of calcitonin
that is released into the circulation in response
to severe systemic inflammation due to bacterial
infection. A recent meta-analysis showed its clinical
value in diagnosing sepsis in critically ill patients
(an area under the receiver operating characteristic
curve of 0.85).5 The meta-analysis, however, was
limited by substantial heterogeneity across different
studies, a wide range of cut-offs used, absence of a
true reference diagnostic standard, and potential
publication bias. It is noteworthy that PCT can be
falsely elevated in inflammation due to other causes,
such as trauma, rhabdomyolysis, surgery, severe
pancreatitis, autoimmune disorders, cardiogenic
shock, and following prolonged resuscitation. It
cannot be solely relied on to discriminate sepsis from
other causes of inflammation, but a plasma PCT level
of ≥0.5 ng/mL is a helpful adjunct when interpreted
along with additional clinical information and serial
monitoring might have a role in guiding subsequent
antibiotic treatment (see below). In case of doubt,
it is advisable to initiate treatment for sepsis early,
and adjust subsequent management and antibiotics
according to the patient’s clinical progress, results of
investigations, and possibly serial PCT monitoring.
Update on protocolised
management: from early goal-directed
therapy to ProCESS-ARISE-ProMISe
In 2001, Rivers et al6 randomised 263 patients
with severe sepsis or septic shock in an emergency
department (ED) to EGDT or usual care. The
sequential goals of EGDT were central venous
pressure (CVP) achieved by fluid resuscitation,
mean arterial pressure (MAP) with vasopressors,
and central venous oxygen saturation (ScvO2) with
red cell transfusion and dobutamine. The result
was an absolute reduction in in-hospital mortality
of 16%.6 The protocol was incorporated into the
surviving sepsis campaign (SSC) 2004, 2008, and the
2012 Guidelines.7
Over the years, concerns have remained
about the external validity of the original trial,
haemodynamic goals, use of CVP and ScvO2
monitoring, blood transfusion, dobutamine, and
resultant higher costs of its implementation.
Subsequently, three large-scale multicentre
randomised controlled trials were published in
2014-2015: the ProCESS (Protocolized Care for
Early Septic Shock) trial,8 the ARISE (Australasian
Resuscitation in Sepsis Evaluation) trial,9 and the
ProMISe (Protocolised Management in Sepsis)
trial.10 All three studies were negative; there was no
survival benefit using protocolised care compared
with usual care (Table 2).6
8 9 10
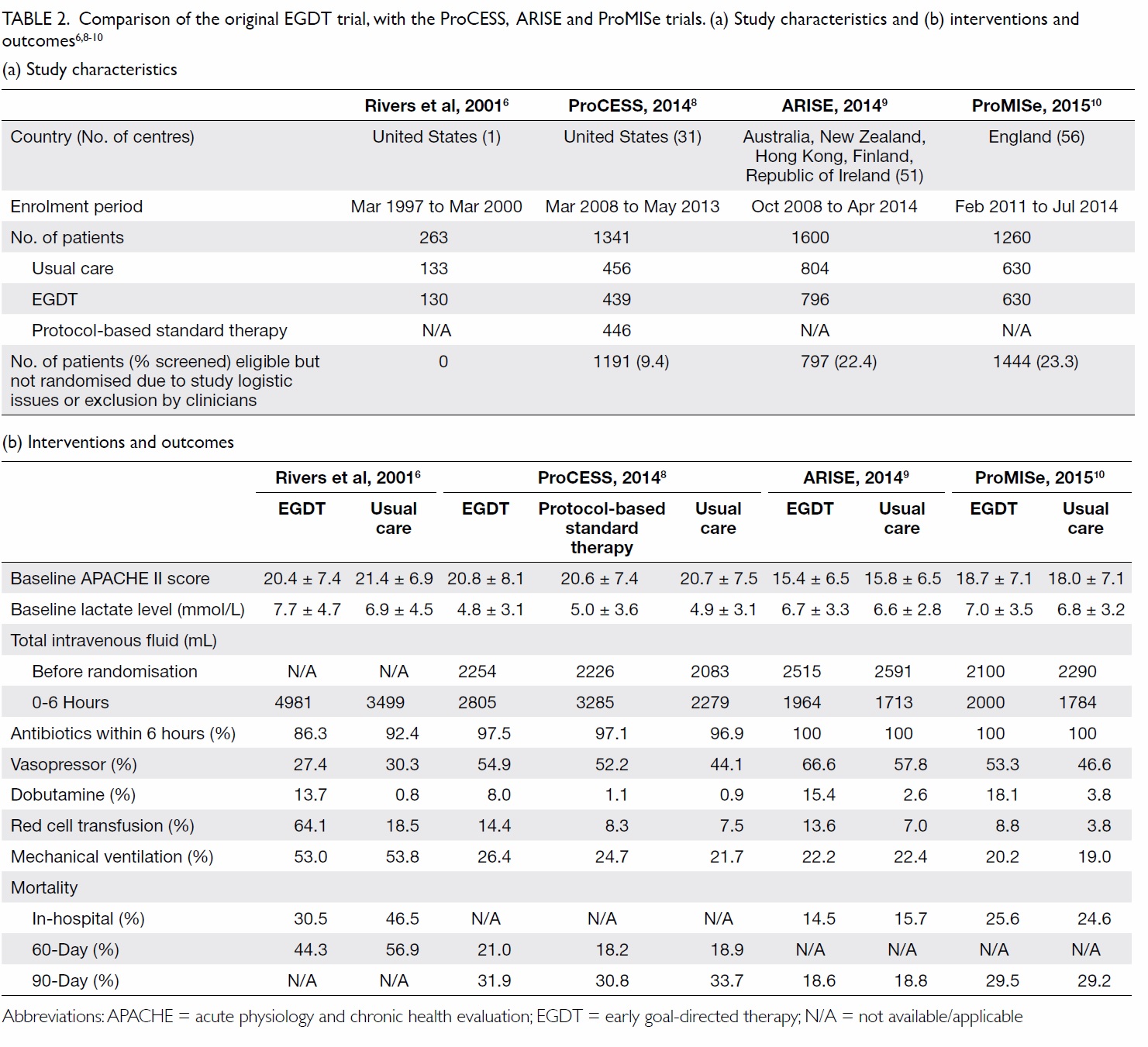
Table 2. Comparison of the original EGDT trial, with the ProCESS, ARISE and ProMISe trials. (a) Study characteristics and (b) interventions and outcomes6 8 9 10
A lack of survival benefit of EGDT in the
latest three trials may be the result of improved
sepsis management since the original trial: nearly
all patients received antibiotics within 6 hours of
presentation, and a significant amount of fluid was
already administered before randomisation (Table
2). Treatment in the usual care groups was guided
by clinical assessment of volume and perfusion
status, and achieved similar mean and systolic blood
pressures at the end of the intervention period.
These trials demonstrated that CVP and ScvO2 goals
confer no additional benefit for sepsis survival.
In response to the new evidence, the SSC
Guidelines updated its 6-hour bundle in April 2015,
and recommended reassessment in the event of
persistent arterial hypotension with either physical
examination or “two of the following (measure CVP,
measure ScvO2, bedside cardiovascular ultrasound,
dynamic assessment of fluid responsiveness with
passive leg raise or fluid challenge)”.11
Clinical management: initial
resuscitation and treatment
Source control
Source control includes drainage of any infected
fluid collection, debridement of infected solid tissue,
and removal of infected foreign bodies or devices.
It should best be achieved within 12 hours of
identification by imaging and/or diagnostic sampling
of the infection foci. Minimally invasive intervention
including percutaneous and endoscopic treatments
should be considered, but surgery is indicated if
control remains inadequate or if there is diagnostic
uncertainty. Damage-control surgery for life-threatening
peritonitis is associated with improved
outcomes.12 It involves an abbreviated initial
laparotomy for haemorrhage and contamination
control, followed by resuscitation before the final
definitive repair and abdominal closure.
Antibiotics
Delay in antimicrobial treatment is associated with
increased mortality, adverse clinical outcome, and
longer intensive care unit (ICU) and hospital stay.13
Effective intravenous antimicrobials should be
initiated as soon as possible after recognition and
within 1 hour for both sepsis and septic shock.14
One study showed that each hour delay reduces
survival by 7.6% in the first 6 hours following the
onset of septic shock.15 Although a recent meta-analysis
pooling data from 11 observational studies
showed no survival benefit of early antibiotic
therapy,16 it failed to analyse all eligible studies
and lacked microbiological considerations. Early
therapy remains logical, especially in patients with
severe infections, although the optimum time frame
for administration remains unknown.17 Delay in
administration can occur anywhere, from ED triage,
making a diagnosis, antibiotic order, drug dispensary
to the actual administration, and should be addressed
with clinical, administrative, and logistics measures
to improve timeliness of treatment.
The choice of initial empirical antimicrobials
should be broad enough to cover the likely pathogens,
while also taking into account recent culture results,
host factors, and susceptibility patterns. The EPIC
II (Extended Prevalence of Infection in Intensive
Care) study showed that in Asia, the common
infective sources are the respiratory system,
abdomen, blood stream, and renal/urinary tract,
while the commonest organisms are Streptococcus
pneumoniae, vancomycin-sensitive Enterococcus,
Klebsiella spp, Pseudomonas spp, and Acinetobacter spp.18 Local microbiologists can regularly provide
antibiotic sensitivity patterns for reference.
Combination therapy, defined as administration
of two or more different classes of antimicrobials with
different mechanisms of action, has the advantages
of broadening the spectrum of coverage, and possible
additive or synergistic effects on pathogens. Meta-analyses
showed that combination antibiotic therapy
improves survival in the most severely ill patients
with septic shock, but may be detrimental to low-risk
patients and increases nephrotoxicity.19 20 Once
the causative pathogens and their susceptibility
patterns are known, de-escalation of antimicrobial
therapy should follow to prevent development of
resistance, as well as reduce drug toxicity and cost.
Discontinuing antibiotics can be considered when
PCT is ≤0.5 ng/mL or serial monitoring shows a
decline of ≥80% of its peak value.21
Appropriate antibiotic dosing to achieve
effective bacterial killing while preventing toxicity
and emergence of resistance is also important. This
is particularly relevant in critically ill patients with
substantial pharmacokinetic variability (Table 3),
who are infected by pathogens with higher minimum
inhibitory concentrations (MIC). Individualised
dosing adjustment requires knowledge of
pharmacokinetic targets and MIC for the organism.
Readers can refer to published reviews for specific
recommendations.22 23 In general, changes to the
volume of distribution will affect initial dosing, while
changes in drug clearance will affect the maintenance
dose. Killing by time-dependent antibiotics (eg β-lactams) correlates with the time fraction when
serum drug concentration exceeds MIC. This can be
achieved by frequent dosing and use of continuous
infusion. Conversely, concentration-dependent
killing (eg aminoglycosides) correlates with the ratio
of peak drug concentration to MIC. A higher dosage
with extended dosing intervals will maximise killing
while minimising toxicity. Due to the complexity and
variability of various factors at play, therapeutic drug
monitoring has been advocated in the critically ill
patients but remains to be available universally.22
23 24
Therapeutic drug monitoring involves direct
measurement of serum antibiotic concentrations
and timely comparison with a therapeutic target to
facilitate adjustments by the clinician or any dosing
software.
Fluid
Type of fluid
Choice of non-blood product can be broadly divided
into crystalloids and colloids. Crystalloids include
normal saline or balanced solutions (eg lactated
Ringer’s solution [B Braun, US], Hartmann’s solution
[Fresenius Kabi, Australia], Plasma-Lyte 148 [Baxter,
US]). Colloids include natural human albumin and
semi-synthetic solutions (gelatin-like Gelofusine [B
Braun, US] or Haemaccel [Sanofi, France], dextran,
and hydroxyethyl starch). Normal saline has a high
chloride content and may produce hyperchloraemic
acidosis and renal vasoconstriction. Balanced
solutions minimise these side-effects by using lactate or acetate as buffers. Weak evidence suggests that
balanced solutions compared with normal saline
reduce acute kidney injury (AKI), the need for
renal replacement therapy (RRT), and mortality in
sepsis.25 26 Nonetheless, the recent SPLIT trial did
not find a difference in AKI among a heterogeneous
group of ICU patients who received a balanced
crystalloid or saline, although the study recruited
predominantly postoperative patients at low risk
who received small doses (median, 2 L) of fluid.27
Colloids theoretically maintain a higher
oncotic pressure and hence intravascular volume
but the CRISTAL (Colloids Versus Crystalloids for
the Resuscitation of the Critically Ill) trial found
no mortality difference among ICU patients with
hypovolaemic shock who were resuscitated with
either colloids or crystalloids.28 Dextran has ceased to
be a resuscitation fluid due to its high anaphylactoid
potential, impact on platelet aggregation with
resultant bleeding complications, and interference
with erythrocyte cross-matching. Gelatins have the
highest risk among the colloids for anaphylactoid
reaction and the lowest intravascular persistence
due to their rapid urinary excretion. Hydroxyethyl
starch is not advisable for acute volume resuscitation
because it deposits in the kidneys, liver, skin and other
tissues, and is associated with increased mortality,
AKI, new-onset hepatic failure, and higher incidences
of pruritus and rash.29 30
31 32 Concerning albumin, the
SAFE (Saline versus Albumin Fluid Evaluation)
trial33 demonstrated no survival benefit among a
general ICU population when either 4% albumin
or normal saline was used for fluid resuscitation,
but predefined subgroup analysis suggested a trend
towards improved survival in patients with severe sepsis who received albumin solution. A decade later,
however, the ALBIOS (Albumin Italian Outcome
Sepsis) study of patients with severe sepsis could not
confirm a survival benefit when albumin was used in
addition to crystalloids compared with crystalloids
alone to maintain a serum albumin level of ≥30 g/L,
although there was a small haemodynamic
advantage and post-hoc subgroup analysis showed a
significantly lower 90-day mortality in patients with
septic shock who received albumin.34
In summary, crystalloids (possibly balanced
solutions) remain the initial fluid of choice in the
resuscitation of sepsis. Routine use of albumin is
not warranted given its higher cost, but it may be
considered in patients with septic shock who do
not respond to crystalloid. There is no evidence
that gelatins are more beneficial, and dextran and
hydroxyethyl starch should be avoided.
Assessment of fluid responsiveness
Both under- and over-hydration can be harmful.35 It
is therefore recommended that 30 mL/kg crystalloid
be given with reassessment of fluid responsiveness
(defined as >10%-15% increase in stroke volume
in response to volume administration) or tissue
perfusion afterwards. Static indices like CVP
and pulmonary capillary wedge pressure are not
good indicators of fluid responsiveness, and are
not recommended for use to guide fluid therapy.
Dynamic indices obtained by inducing a change
in the preload and monitoring the corresponding
change in cardiac output (CO) or its derivatives
should be used instead (Table 4).
An arterial waveform pulse pressure variation
(PPV) of >13%, induced by heart-lung interactions during mechanical ventilation, predicts fluid
responsiveness with a high degree of accuracy in
controlled settings.36 37 Its accuracy, however, is
lowered by arrhythmia, spontaneous breathing
activity, low-tidal-volume ventilation (<8 mL/kg
ideal body weight), low heart-rate-to-respiratory-rate
ratio (<3.6), and right ventricular dysfunction
(peak systolic velocity of tricuspid annulus <0.15
m/s). Raised intra-abdominal pressure (IAP)
exaggerates PPV, and one study found that PPV/IAP of <1.41 could identify false-positive patients.38
These confounders limit the application of PPV
in routine clinical practice, in particular during
protective ventilation for acute respiratory distress
syndrome (ARDS).
A virtual fluid challenge test with passive leg
raising can avoid the above caveats as it does not
rely on mechanical ventilation to induce changes
in preload and, unlike other methods, has been
validated in patients with breathing efforts and
arrhythmia. When coupled with CO monitoring,
passive leg raising has an excellent predictive
accuracy.39 Accuracy drops when arterial pulse
pressure instead of CO is used, as well as in patients
with raised IAP of ≥16 mm Hg.40
A mini-fluid challenge (100 mL infused rapidly
over 1 minute) is an alternative method and limits
cumulative positive fluid balance in non-responders. Unlike PPV, it remains accurate at times of low-tidal-volume ventilation.41
Respiratory variation of the inferior vena
cava diameter is another accurate marker of fluid
responsiveness in patients who are mechanically
ventilated,42 but its use in spontaneously breathing
patients is more controversial.
Vasopressors
Guidelines of SSC recommend maintaining MAP at
a minimal of 65 mm Hg.14 The SEPSISPAM (Sepsis
and Mean Arterial Pressure) Investigators studied
776 septic shock patients, and found that targeting
a MAP of 80-85 mm Hg rather than 65-70 mm Hg
did not result in any difference in 28- or 90-day
mortality.43 A vasopressor should be considered
when MAP of ≥65 mm Hg cannot be maintained
despite adequate fluid resuscitation.
For the choice of vasopressors, studies
that compared norepinephrine (with or without
additional dobutamine in patients with low CO)
with epinephrine found no difference in all-cause
28-day mortality,44 or the time to achievement of a
clinician-prescribed MAP goal.45 Epinephrine use
was associated with significant tachycardia and
lactic acidosis that did not affect haemodynamic
stabilisation or survival. The hyperlactataemia
represents exaggerated aerobic glycolysis instead of
ongoing tissue hypoxia, but potentially interferes
with interpretation of serial serum lactate
measurements. The 2008 VASST (Vasopressin and
Septic Shock Trial)46 randomised 779 septic shock
patients to receive either norepinephrine alone or
norepinephrine plus low-dose vasopressin (0.03
U/min), and found no difference in all-cause 28-day
mortality. The SOAP II Trial randomised 1679
patients with shock to receive either norepinephrine
or dopamine, and found no difference in all-cause
28-day mortality but a significantly higher rate of
arrhythmias in the dopamine group (the number
needed to harm was 9). In their subgroup analysis,
dopamine was associated with higher mortality in
cardiogenic shock, but not septic and hypovolaemic
shock.47
Norepinephrine is therefore recommended
as the first-line vasopressor for septic shock.
In refractory hypotension, epinephrine or low-dose
vasopressin (0.03 units/min) may be added.
Dopamine should be avoided except in highly
selected patients who are bradycardic and at low risk
of tachyarrhythmias.
Resuscitation endpoints
The optimal goal for sepsis resuscitation remains
unknown. While under resuscitation is detrimental,
achieving supranormal targets has also been shown
to cause harm.48 The MAP (perfusion pressure)
of ≥65 mm Hg and urine output of ≥0.5 mL/kg/h
are the recommended targets. The EMShockNet
Trial showed that there was no difference in
hospital mortality when using lactate clearance
(>10%) or ScvO2 (>70%) as goals of early sepsis
resuscitation.49 Hyperlactataemia in sepsis, however,
can result from increased production driven by
endogenous or exogenous epinephrine-stimulated
aerobic glycolysis, endotoxin inhibition of pyruvate
dehydrogenase, and decreased lactate metabolism
due to liver and renal dysfunction. Thus, persistent
hyperlactataemia does not necessarily indicate
anaerobic metabolism and tissue hypoxia, and should
not be solely relied on to guide therapy that aims to
boost oxygen delivery in patients who are otherwise
clinically improving. Conversely, normalisation of
serum lactate is reassuring as it is associated with
reduced hospital mortality in critically ill patients.50
Adjunctive therapy
Blood transfusion
In the original EGDT protocols, once ScvO2
drops below 70%, blood transfusion to achieve a
haematocrit level of ≥30% was recommended to
boost oxygen delivery. The 1999 TRICC (Transfusion
Requirements in Critical Care) trial demonstrated
lower rates of in-hospital mortality with a restrictive
rather than liberal transfusion strategy. This trial, however, excluded septic shock patients. The 2014
TRISS (Transfusion Requirements in Septic Shock)
trial randomised 998 septic shock patients to either a
liberal blood transfusion strategy with a transfusion
threshold of haemoglobin of ≤90 g/L or a restrictive
strategy with a threshold of ≤70 g/L.51 Mortality at 90
days, rate of ischaemic events, and use of life support
were similar. A transfusion threshold of 70 g/L is
therefore recommended. For patients with ongoing
acute coronary syndrome or chronic cardiovascular
disease, targeting a higher haemoglobin level of 100 g/L
might be beneficial but remains to be proven.52
Glucocorticoids
Glucocorticoids have anti-inflammatory and
immunosuppressive effects. Despite the positive
Annane Trial in 2002, the subsequently larger
multicentre CORTICUS (Corticosteroid Therapy of
Septic Shock) Trial53 was negative. It randomised 499
patients with septic shock to receive 6-hourly 50-mg
hydrocortisone or placebo, with the dose tapered
over 11 days. Hydrocortisone did not improve 28-day
survival in patients with septic shock, and should not
be routinely used for septic shock before adequate
fluid resuscitation and vasopressor therapy.14 If used
in refractory shock, early administration within 9
hours of commencement of vasopressor is advised.54
Glucose control
Tight glycaemic control (blood glucose, 4.4-6.1 mmol/L)
was once commonly practised after the 2001
Leuven Surgical Trial. In 2009, the NICE-SUGAR
(Normoglycemia in Intensive Care Evaluation—Surviving Using Glucose Algorithm Regulation)
study randomised 6104 ICU patients, and showed
that intensive glucose control (4.5-6.0 mmol/L)
increased mortality compared with a target of <10
mmol/L.55 Post-hoc analysis further demonstrated
an association between hypoglycaemia and
an increased risk of death in a dose-response
relationship. This association was strongest for
death from distributive, including septic shock.56
Guidelines of SSC recommend targeting an upper
blood glucose level of <10 mmol/L to reduce the risk
of hypoglycaemia.14
Organ support
Kidney
The optimal timing of RRT in the absence of overt
life-threatening complications (severe metabolic
acidosis, hyperkalaemia, and/or fluid overload) is
uncertain. Prior studies as well as the recent ELAIN
(Early vs Late Initiation of Renal Replacement
Therapy in Critically Ill Patients With Acute Kidney
Injury57) and AKIKI (Artificial Kidney Initiation in
Kidney Injury58) trials have yielded contradictory
results, partly because of the heterogeneous
definitions of ‘early’ and ‘late’ initiation of RRT. It
is hoped that the upcoming IDEAL-ICU (Initiation
of Dialysis Early versus Delayed in Intensive
Care Unit59) and STARRT-AKI (Standard versus
accelerated initiation of renal replacement therapy
in acute kidney injury60) trials will provide more
evidence on the subject. Regarding the intensity of
renal support in critically ill patients with AKI, an
effluent rate of 25 mL/kg/h is considered adequate
and high-volume haemofiltration is not superior.61
62
Survival benefit of blood purification strategies has
yet to be proven.
Lungs
Of note, ARDS is a frequent complication of sepsis.
Optimal ventilatory support prevents further lung
injury and the resultant biotrauma from cytokine
release. A lung protective strategy with low tidal
volume (6 mL/kg ideal body weight) remains the
cornerstone of treatment.63 A higher positive end-expiratory
pressure should be reserved for patients
with moderate-to-severe ARDS as defined by the
latest Berlin definition.64 Early (intubated for <36
hours) and sustained (≥16 consecutive hours per
day) prone positioning in moderate-to-severe ARDS
has proven survival advantage when practised in
conjunction with lung protective ventilation.65
Conclusion
Optimal sepsis management involves both refinement
of clinical interventions and administrative logistics
for the timeliness of their delivery. Early recognition
of sepsis, timely source control, prompt and effective
antibiotic administration at the right dose, immediate
fluid resuscitation as guided by bedside reassessment,
and dynamic indices of fluid responsiveness remain
the mainstay of sepsis management.
Declaration
All authors have disclosed no conflicts of interest.
References
1. Gaieski DF, Edwards JM, Kallan MJ, Carr BG. Benchmarking
the incidence and mortality of severe sepsis in the United
States. Crit Care Med 2013;41:1167-74.
Crossref
2. Kaukonen KM, Bailey M, Pilcher D, Cooper DJ, Bellomo
R. Systemic inflammatory response syndrome criteria in
defining severe sepsis. N Engl J Med 2015;372:1629-38.
Crossref
3. Bone RC, Balk RA, Cerra FB, et al. Definitions for sepsis
and organ failure and guidelines for the use of innovative
therapies in sepsis. The ACCP/SCCM Consensus
Conference Committee. American College of Chest
Physicians/Society of Critical Care Medicine. Chest
1992;101:1644-55. Crossref
4. Singer M, Deutschman CS, Seymour CW, et al. The Third
International Consensus Definitions for Sepsis and Septic
Shock (Sepsis-3). JAMA 2016;315:801-10.
Crossref
5. Wacker C, Prkno A, Brunkhorst FM, Schlattmann P. Procalcitonin as a diagnostic marker for sepsis: a systematic
review and meta-analysis. Lancet Infect Dis 2013;13:426-35. Crossref
6. Rivers E, Nguyen B, Havstad S, et al. Early goal-directed
therapy in the treatment of severe sepsis and septic shock.
N Engl J Med 2001;345:1368-77.
Crossref
7. Dellinger RP, Levy MM, Rhodes A, et al. Surviving sepsis
campaign: international guidelines for management
of severe sepsis and septic shock: 2012. Crit Care Med
2013;41:580-637.
Crossref
8. ProCESS Investigators, Yealy DM, Kellum JA, et al. A
randomized trial of protocol-based care for early septic
shock. N Engl J Med 2014;370:1683-93.
Crossref
9. ARISE Investigators; ANZICS Clinical Trial Group, Peake
SL, Delany A, et al. Goal-directed resuscitation for patients
with early septic shock. N Engl J Med 2014;371:1496-506.
Crossref
10. Mouncey PA, Osborn TM, Power GS, et al. Trial of early,
goal-directed resuscitation for septic shock. N Engl J Med
2015;372:1301-11.
Crossref
11. Surviving Sepsis Campaign. Updated bundles in response to
new evidence. Available from: http://www.survivingsepsis.org/SiteCollectionDocuments/SSC_Bundle.pdf. Accessed
5 Jun 2016.
12. Waibel BH, Rotondo MF. Damage control for intra-abdominal
sepsis. Surg Clin North Am 2012;92:243-57.
Crossref
13. Ferrer R, Martin-Loeches I, Phillips G, et al. Empirical
antibiotic treatment reduces mortality in severe sepsis and
septic shock from the first hour: results from a guideline-based
performance improvement program. Crit Care Med
2014;42:1749-55.
Crossref
14. Rhodes A, Evans LE, Alhazzani W, et al. Surviving Sepsis
Campaign: International Guidelines for Management of
Sepsis and Septic Shock: 2016. Crit Care Med 2017;45:486-552. Crossref
15. Kumar A, Roberts D, Wood KE, et al. Duration of
hypotension before initiation of effective antimicrobial
therapy is the critical determinant of survival in human
septic shock. Crit Care Med 2006;34:1589-96.
Crossref
16. Sterling SA, Miller WR, Pryor J, Puskarich MA, Jones AE.
The impact of timing of antibiotics on outcomes in severe
sepsis and septic shock: a systematic review and meta-analysis.
Crit Care Med 2015;43:1907-15.
Crossref
17. Almeida M, Ribeiro O, Aragão I, et al. Differences
in compliance with Surviving Sepsis Campaign
recommendations according to hospital entrance time: day
versus night. Crit Care 2013;17:R79.
Crossref
18. Vincent JL, Rello J, Marshall J, et al. International study of
the prevalence and outcomes of infection in intensive care
units. JAMA 2009;302:2323-9.
Crossref
19. Kumar A, Safdar N, Kethireddy S, Chateau D. A survival
benefit of combination antibiotic therapy for serious
infections associated with sepsis and septic shock is
contingent only on the risk of death: a meta-analytic/meta-regression
study. Crit Care Med 2010;38:1651-64.
Crossref
20. Paul M, Benuri-Silbiger I, Soares-Weiser K, Leibovici
L. Beta lactam monotherapy versus beta lactam-aminoglycoside
combination therapy for sepsis in
immunocompetent patients: systematic review and meta-analysis
of randomised trials. BMJ 2004;328:668.
Crossref
21. de Jong E, van Oers JA, Beishuizen A, et al. Efficacy and
safety of procalcitonin guidance in reducing the duration of
antibiotic treatment in critically ill patients: a randomised,
controlled, open-label trial. Lancet Infect Dis 2016;16:819-27.
Crossref
22. Jamal JA, Economou CJ, Lipman J, Roberts JA. Improving
antibiotic dosing in special situations in the ICU: burns,
renal replacement therapy and extracorporeal membrane
oxygenation. Curr Opin Crit Care 2012;18:460-71.
Crossref
23. Choi G, Gomersall CD, Tian Q, Joynt GM, Li AM, Lipman
J. Principles of antibacterial dosing in continuous renal
replacement therapy. Blood Purif 2010;30:195-212.
Crossref
24. Roberts JA, Abdul-Aziz MH, Lipman J, et al. Individualised
antibiotic dosing for patients who are critically ill:
challenges and potential solutions. Lancet Infect Dis
2014;14:498-509.
Crossref
25. Yunos NM, Bellomo R, Hegarty C, Story D, Ho L, Bailey
M. Association between a chloride-liberal vs chloride-restrictive
intravenous fluid administration strategy and
kidney injury in critically ill adults. JAMA 2012;308:1566-72. Crossref
26. Rochwerg B, Alhazzani W, Sindi A, et al. Fluid resuscitation
in sepsis: a systematic review and network meta-analysis.
Ann Intern Med 2014;161:347-55.
Crossref
27. Young P, Bailey M, Beasley R, et al. Effect of a buffered
crystalloid solution vs saline on acute kidney injury among
patients in the intensive care unit: The SPLIT Randomized
Clinical Trial. JAMA 2015;314:1701-10.
Crossref
28. Annane D, Siami S, Jaber S, et al. Effects of fluid
resuscitation with colloids vs crystalloids on mortality in
critically ill patients presenting with hypovolemic shock:
the CRISTAL randomized trial. JAMA 2013;310:1809-17.
Crossref
29. Brunkhorst FM, Engel C, Bloos F, et al. Intensive insulin
therapy and pentastarch resuscitation in severe sepsis. N
Engl J Med 2008;358:125-39.
Crossref
30. Perner A, Haase N, Guttormsen AB, et al. Hydroxyethyl
starch 130/0.42 versus Ringer’s acetate in severe sepsis. N
Engl J Med 2012;367:124-34.
Crossref
31. Myburgh JA, Finfer S, Bellomo R, et al. Hydroxyethyl
starch or saline for fluid resuscitation in intensive care. N
Engl J Med 2012;367:1901-11.
Crossref
32. Guidet B, Martinet O, Boulain T, et al. Assessment of
hemodynamic efficacy and safety of 6% hydroxyethylstarch
130/0.4 vs. 0.9% NaCl fluid replacement in patients
with severe sepsis: the CRYSTMAS study. Crit Care
2012;16:R94. Crossref
33. Finfer S, Bellomo R, Boyce N, et al. A comparison of
albumin and saline for fluid resuscitation in the intensive
care unit. N Engl J Med 2004;350:2247-56.
Crossref
34. Caironi P, Tognoni G, Masson S, et al. Albumin replacement
in patients with severe sepsis or septic shock. N Engl J Med
2014;370:1412-21.
Crossref
35. Boyd JH, Forbes J, Nakada TA, Walley KR, Russell JA.
Fluid resuscitation in septic shock: a positive fluid balance
and elevated central venous pressure are associated with
increased mortality. Crit Care Med 2011;39:259-65.
Crossref
36. Marik PE, Cavallazzi R, Vasu T, Hirani A. Dynamic
changes in arterial waveform derived variables and fluid
responsiveness in mechanically ventilated patients:
a systematic review of the literature. Crit Care Med
2009;37:2642-7.
Crossref
37. Michard F, Boussat S, Chemla D, et al. Relation between
respiratory changes in arterial pulse pressure and fluid
responsiveness in septic patients with acute circulatory
failure. Am J Respir Crit Care Med 2000;162:134-8.
Crossref
38. Royer P, Bendjelid K, Valentino R, Résière D, Chabartier
C, Mehdaoui H. Influence of intra-abdominal pressure on
the specificity of pulse pressure variations to predict fluid
responsiveness. J Trauma Acute Care Surg 2015;78:994-9.
Crossref
39. Monnet X, Marik P, Teboul JL. Passive leg raising for
predicting fluid responsiveness: a systematic review and
meta-analysis. Intensive Care Med 2016;42:1935-47.
Crossref
40. Mahjoub Y, Touzeau J, Airapetian N, et al. The passive
leg-raising maneuver cannot accurately predict fluid
responsiveness in patients with intra-abdominal
hypertension. Crit Care Med 2010;38:1824-9.
Crossref
41. Mallat J, Meddour M, Durville E, et al. Decrease in pulse
pressure and stroke volume variations after mini-fluid
challenge accurately predicts fluid responsiveness. Br J
Anaesth 2015;115:449-56.
Crossref
42. Barbier C, Loubières Y, Schmit C, et al. Respiratory changes
in inferior vena cava diameter are helpful in predicting
fluid responsiveness in ventilated septic patients. Intensive
Care Med 2004;30:1740-6.
Crossref
43. Asfar P, Meziani F, Hamel JF, et al. High versus low blood-pressure
target in patients with septic shock. N Engl J Med
2014;370:1583-93.
Crossref
44. Annane D, Vignon P, Renault A, et al. Norepinephrine plus
dobutamine versus epinephrine alone for management of
septic shock: A randomised trial. Lancet 2007;370:676-84.
Crossref
45. Myburgh JA, Higgins A, Jovanovska A, et al. A comparison
of epinephrine and norepinephrine in critically ill patients.
Intensive Care Med 2008;34:2226-34.
Crossref
46. Russell JA, Walley KR, Singer J, et al. Vasopressin versus
norepinephrine infusion in patients with septic shock. N
Engl J Med 2008;358:877-87.
Crossref
47. De Backer D, Biston P, Devriendt J, et al. Comparison of
dopamine and norepinephrine in the treatment of shock.
N Engl J Med 2010;362:779-89.
Crossref
48. Hayes MA, Timmins AC, Yau EH, Palazzo M, Hinds
CJ, Watson D. Elevation of systemic oxygen delivery
in the treatment of critically ill patients. N Engl J Med
1994;330:1717-22.
Crossref
49. Jones AE, Shapiro NI, Trzeciak S, et al. Lactate clearance
vs central venous oxygen saturation as goals of early sepsis
therapy: a randomized clinical trial. JAMA 2010;303:739-46.
Crossref
50. Nichol A, Bailey M, Egi M, et al. Dynamic lactate indices
as predictors of outcome in critically ill patients. Crit Care
2011;15:R242. Crossref
51. Holst LB, Haase N, Wetterslev J, et al. Lower versus higher
hemoglobin threshold for transfusion in septic shock. N
Engl J Med 2014;371:1381-91.
Crossref
52. Docherty AB, O’Donnell R, Brunskill S, et al. Effect of
restrictive versus liberal transfusion strategies on outcomes
in patients with cardiovascular disease in a non-cardiac
surgery setting: systematic review and meta-analysis. BMJ
2016;352:i1351. Crossref
53. Sprung CL, Annane D, Keh D, et al. Hydrocortisone therapy
for patients with septic shock. N Engl J Med 2008;358:111-24. Crossref
54. Katsenos CS, Antonopoulou AN, Apostolidou EN, et al.
Early administration of hydrocortisone replacement after
the advent of septic shock: impact on survival and immune
response. Crit Care Med 2014;42:1651-7.
Crossref
55. NICE-SUGAR Study Investigators, Finfer S, Chittock DR,
et al. Intensive versus conventional glucose control in
critically ill patients. N Engl J Med 2009;360:1283-97.
Crossref
56. NICE-SUGAR Study Investigators, Finfer S, Liu B, et al.
Hypoglycemia and risk of death in critically ill patients. N Engl J Med 2012;367:1108-18.
Crossref
57. Zarbock A, Kellum JA, Schmidt C, et al. Effect of early vs
delayed initiation of renal replacement therapy on mortality
in critically ill patients with acute kidney injury: the ELAIN
randomized clinical trial. JAMA 2016;315:2190-9.
Crossref
58. Gaudry S, Hajage D, Schortgen F, et al. Initiation strategies
for renal-replacement therapy in the Intensive Care Unit.
N Engl J Med 2016;375:122-33.
Crossref
59. Barbar SD, Binquet C, Monchi M, Bruyère R, Quenot JP.
Impact on mortality of the timing of renal replacement
therapy in patients with severe acute kidney injury in septic
shock: the IDEAL-ICU study (initiation of dialysis early
versus delayed in the intensive care unit): study protocol
for a randomized controlled trial. Trials 2014;15:270.
Crossref
60. Wald R, Adhikari NK, Smith OM, et al. Comparison of
standard and accelerated initiation of renal replacement
therapy in acute kidney injury. Kidney Int 2015;88:897-904.
Crossref
61. RENAL Replacement Therapy Study Investigators,
Bellomo R, Cass A, et al. Intensity of continuous renal-replacement
therapy in critically ill patients. N Engl J Med
2009;361:1627-38.
Crossref
62. Joannes-Boyau O, Honoré PM, Perez P, et al. High-volume
versus standard-volume haemofiltration for septic shock
patients with acute kidney injury (IVOIRE study): a
multicentre randomized controlled trial. Intensive Care
Med 2013;39:1535-46.
Crossref
63. Ventilation with lower tidal volumes as compared with
traditional tidal volumes for acute lung injury and the acute
respiratory distress syndrome. The Acute Respiratory
Distress Syndrome Network. N Engl J Med 2000;342:1301-8.
Crossref
64. Briel M, Meade M, Mercat A, et al. Higher vs lower positive
end-expiratory pressure in patients with acute lung injury
and acute respiratory distress syndrome: systematic review
and meta-analysis. JAMA 2010;303:865-73.
Crossref
65. Guérin C, Reignier J, Richard JC, et al. Prone positioning in
severe acute respiratory distress syndrome. N Engl J Med
2013;368:2159-68.
Crossref